Manufacturing and Evaluation of High-Quality Composites using Out-of-Autoclave Prepreg - Juniper Publishers
Academic Journal of Polymer Science - Juniper Publishers
Abstract
Carbon fiber-reinforced thermoset polymers have become popular in a wide variety of applications such as primary aerospace structures, sporting goods and wind energy systems. Autoclave processing has been the preferred method for fabricating high performance composites. However, the need for low-cost, high-performance composites prompted researchers and industries to develop new techniques such as vacuum aided resin transfer molding (VARTM) and vacuum-bag-only cure out-of-autoclave (OOA). Manufacturing parts with less than 1% void content, on the other hand, remains a difficulty. In the present study, the OOA technique was used to create high-quality (less than 0.25 percent void content) carbon/epoxy composites. The phases in the processing that result in good quality are described. Physical, mechanical, and fatigue properties of the manufactured composites were evaluated.
Keywords: Fiber-reinforced; Polymers; Carbon; Composites; Vacuum
Introduction
In spite of numerous application possibilities, the usage of composites has been limited because of high costs. While the material costs sum to 8-10% of the total costs, manufacturing and processing costs contribute to the majority of the overall costs of the composites [1]. Cost savings of up to 75% have been achieved by using low-cost composite manufacturing techniques and by making integral parts [2]. A reduction in man-hours by 70-85% was also reported when implementing automated composite tape layers [3]. Hence, several studies have been devoted over the past few decades in developing non-autoclave manufacturing techniques that can significantly reduce the manufacturing costs of composites [4-10]. Bond et al. [11] presented a comparative summary of the physical, mechanical, and thermal performance of composites manufactured using different non-autoclave processes developed in the past few decades. In addition to huge capital and tool cost-savings, non-autoclave composite manufacturing processes offer several advantages such as scalability to large parts, and flexibility to manufacture hybrid, complex-shaped parts [5]. The out-of-autoclave (OOA) process is a vacuum-bag-only cure process that uses engineered prepregs that can be cured in regular ovens instead of an autoclave. Centea et al. [12] conducted a literature review on the processing of vacuum- bag-only prepreg and their effect on composite quality. They also presented the development and defining properties of vacuum bag only prepreg. The cost and environmental performance are also discussed in their study. The OOA process not only results in less energy consumption but the lower capital and tooling costs, fewer coefficient of thermal expansion issues, and the scalability to larger and integral parts made it a competitive alternative to the autoclave process. Developing low-cost advanced composites will allow to fully utilize the advantages of composites and to advance the usage of composites in several applications. And the improved performance of the composites is directly related to the fiber, resin, and especially void content. While void content less than 2% is typically desirable in aerospace composites, OOA has to produce composites with less than 1% to truly deliver advanced composite products that are comparable to autoclave composites.
Park et al. [13] utilized vacuum-bag-only to manufacture carbon/epoxy composites and investigated curing techniques for producing high-performance composites with low void content. They stated that improving the resin flow may allow for producing parts with minimal void content (1.3%). The composite laminates generated by their recommended technique showed a slight decrease in compressive strength compared to autoclave curing.
The compressive strength decreased by 6.5% for [0/90]₄ stacking sequence and 7.6% for [0/452/90]s stacking sequence. The inplane shear strength increased by 3% compared to laminates obtained by autoclave curing. In the present study, high-quality composites with a void content of less than 0.25% have been consistently manufactured employing the OOA manufacturing process. The manufacturing process utilized in accomplishing the high-quality composites is presented. Physical, mechanical, and fatigue tests have been conducted to evaluate the performance of the manufactured composites. Low-velocity impact tests were performed on the manufactured composite panels. Residual compressive strength of the impacted panels was evaluated. The effect of impact on the fatigue life of the composites was studied.
Materials
MTM45-1/CF2412 carbon prepregs obtained from Cytec Engineered Materials Inc., NJ, USA have been used for the present study. These prepregs contain 6K 5HS AS4C carbon fabric impregnated with MTM 45-1, a variable cure temperature, highperformance toughened epoxy resin.
Manufacturing
Flat composite panels have been manufactured using the OOA manufacturing process. The schematic of the bagging procedure employed for the OOA process is shown in Figure 1. The manufacturing procedure includes laying up the prepregs that were cut to the required dimensions and orientations onto an aluminum mold free from surface defects and already coated with Frekote release agent. Hand pressure and rollers were used to press the prepregs over the mold starting from one side of the prepreg and moving progressively towards the rest of the surface. This process is repeated for all the prepregs to remove entrapped air bubbles as well as folds or wrinkles. Thin glass strings, FEP release film, breather, and vacuum outlets were placed and sealed with a vacuum bag. A vacuum line was connected to the vacuum pump and checked for any leaks. A two-stage vacuum pump with a capacity of 5 L s-1and an ultimate vacuum of 0.013 Pa has been used to manufacture these panels. The set-up was maintained under vacuum for 12 hours. The lay-up is heated to 180oF and held for 4.5 hours. The temperature is then increased to 250oF and held for 4.5 hrs. The part is then cooled down to room temperature, demolded, and post-cured at 350oF for 2 hrs.
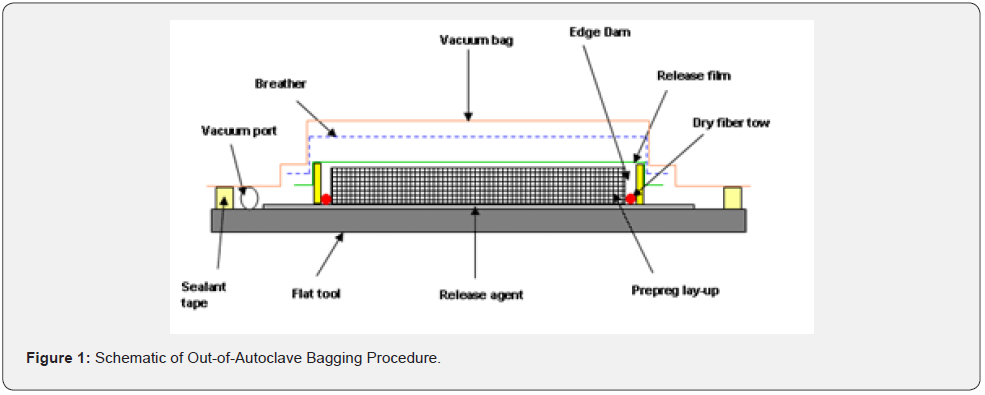
Characterization
Fiber volume fraction testing using sulphuric acid digestion method
Fiber volume fraction tests were conducted on the manufactured OOA composite panels using sulphuric acid digestion method. Four specimens each weighing from 0.50 to 2 gm. were cut from the panel. The edges of the specimens were polished thoroughly to facilitate accurate density measurements. The samples were dried in an oven for 1 hour at 120°C to remove any surface moisture, weighed, and tested for density. Table 1 shows the density, fiber volume fraction, and void content of the composite samples. The samples had an average fiber volume fraction of 53.99 %, and void content of 0.21 %. According to published studies, the amount of voids in a material has a direct impact on its mechanical properties [13-15]. For interlaminar shear strength, flexural strength, and flexural modulus, Ghiorse et al. reported reductions of 9.7%, 10.3%, and 5.3 percent per percent void, respectively [14]. Sergio et al. discovered that increasing void content had a significant negative impact on the fatigue life of composite constructions [15]. As a result, lowering the void percentage from 1% to around 0.25 percent should improve mechanical qualities.
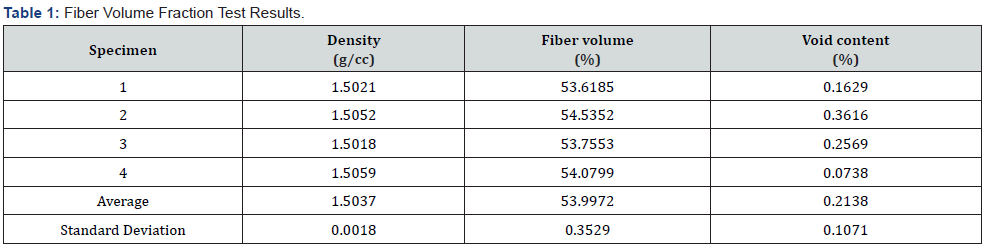
Tensile Tests
Static tensile tests were performed on the OOA composites to evaluate the ultimate tensile strength required for fatigue testing. Samples of 2.286 mm (0.09 in.) thickness (6 layers) with 25.4 mm (1 in.) and 12.7 mm (0.5 in.) width either slipped or failed in the grips. Hence the thickness of the samples was decreased to 0.064 in (4 layers). While samples with 25.4 mm (1 in.) width failed in the grips without slipping, 12.7 mm (0.5 in.) wide samples failed in the middle. The test results obtained for 12.7 mm (0.5 in.) width and 1.626 mm (0.064 in.) thickness were reported below. Composites coupons were cut from the panels were manufactured using 4 layers of MTM45-1/CF2412 OOA prepregs. Tests were conducted on the coupons using Instron 4204 testing machine in accordance with ASTM D 3039. Samples were tested at a crosshead speed of 12.7 mm/min. (0.05 in./min). The ultimate tensile strength, modulus, and failure strain are tabulated in Table 2. The samples had an average tensile modulus, strength, and strain to failure of 824.79 MPa, 65.20 GPa, and 1.27% respectively. Hence the post-impact fatigue tension tests were performed at three stress levels – 50% Sult = 413.68 MPa, 65% Sult = 53.78 MPa and 75% Sult = 62.05 MPa (Sult -ultimate strength). When the samples fail at these levels during the fatigue tests, the stress level values were further dropped down.

Flexure Tests
Static flexure tests were performed on the OOA composites to evaluate the bending properties. Samples of 0.09 in thickness (6 layers) with 12.7 mm (0.5 in.) width manufactured from 6 layers of MTM45-1/CF2412 prepregs were used as test specimens. Tests were conducted on an Instron testing machine according to ASTM D790-03. A span to depth ratio of 40:1 was used to avoid failure by shear. Six specimens were tested at a crosshead speed of 6.096 mm/ min. (0.24 in./min.) The ultimate flexural strength, modulus, and strain to failure values are tabulated in Table 3.

Low-Velocity Impact Tests
Low-velocity impact tests have been performed on the composite panels manufactured using Out-of-Autoclave (OOA) process. A Dynatup Instron Model 9250 Impact Testing Machine with impulse control and a data system was used to carry out the low-velocity impact tests. Three different energy levels of 10J, 20J, and 25J were considered. The hemi-spherical impactor had a mass of 6.88 Kg and a diameter of 12.7 mm (0.5 in). The energytime history, load vs. displacement, and velocity-time history plots are shown in Figures 2 & 4 respectively. The impactor penetrated the samples at 30J of energy.
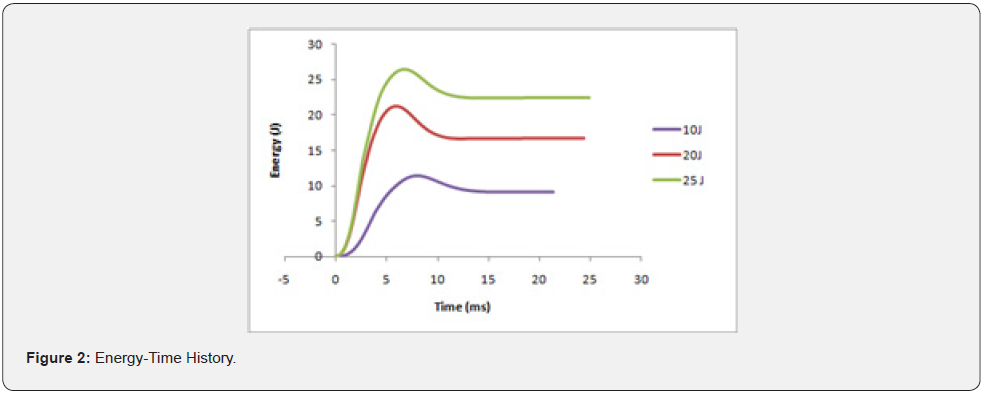

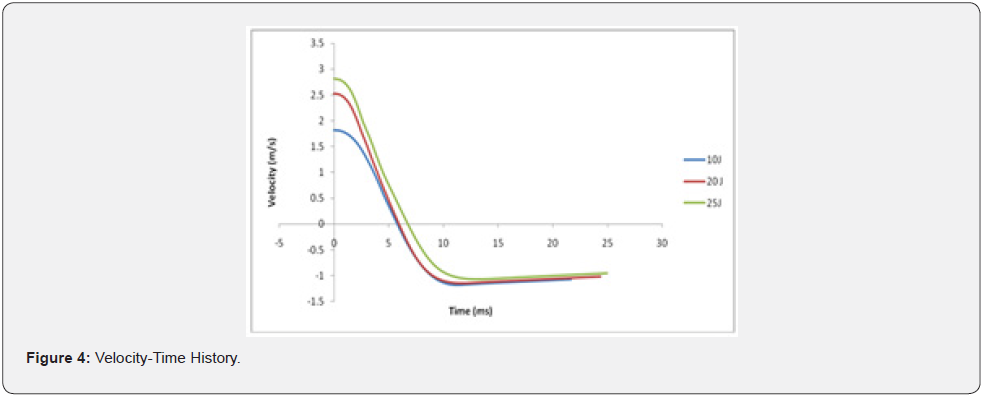
Compression-After-Impact (CAI) Tests
CAI tests have been conducted on MTM45-1/CF2412 composites manufactured using the OOA process. The tests were performed according to ASTM D7137. Four specimens of size 152.4 mm (6 in.) x 152.4 mm (6 in.) were first subjected to low-velocity impact tests and then machined to 152.4 mm (6 in.) x 101.6 mm (4 in.) for the CAI tests. Laminate construction consists of 12 fabric plies with a stacking sequence of [(+45/-45)/(0/90)]3S. Impact energy per unit thickness of 6672 J/m, an industry standard for evaluating thick, quasi-isotropic laminates was selected. Just clearly visible impact damage (VID) has been observed at 32J. The CAI test fixture is edge-loaded between the flat platens. Loads were applied at a cross-head speed of 1.27 mm/min. (0.05 in./ min). Compression load vs. deflection curves are shown in Figure 5. The ultimate compression-after-impact strength values of the specimens are tabulated in Table 4. The front view of the tested samples is shown in Figure 6.
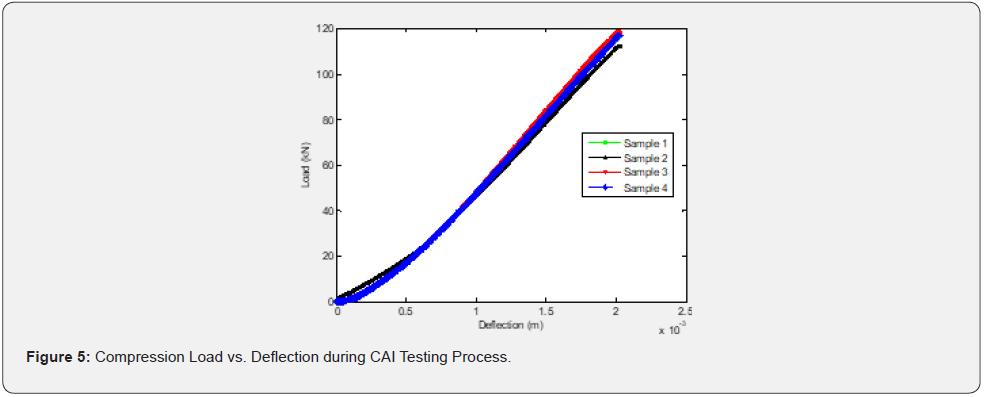

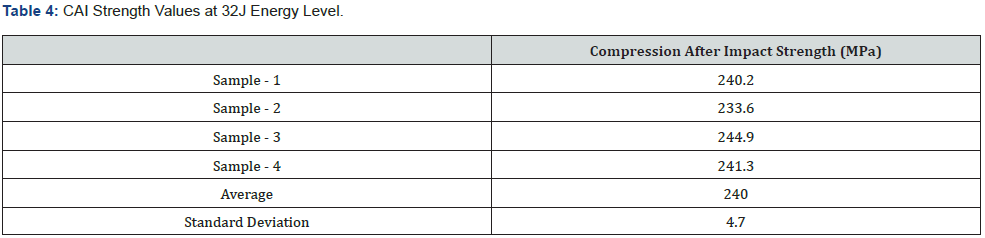
Tension Fatigue Tests
Fatigue tests have been conducted on unimpacted OOA composites in an MTS 810 closed-loop servo-hydraulic testing system. Tests were performed on 12.7 mm (0.5 in.) wide x 254 mm (10 in.) long x 1.6256 mm (0.064 in.) thick MTM45-1/CF2412 samples. Fatigue behavior of the coupons at sinusoidal tension – tension loadings of 80%, 85%, 86%, 88%, and 90% of the ultimate strength (827.37 MPa) or ultimate tensile load of 15.57 kN (3500 lb) have been observed. A frequency of 2Hz and a load ratio of R = 0.1 (R = σmin/ σmax) have been used. Failure of samples in the grips was not observed with an increase in grip pressure to 5.75 MPa. The fatigue life of the coupons at different loadings is presented in Table 5. Figure 7 shows the S-N curve of an unimpacted sample under tension–tension (T-T) loading. Since the gap between the fatigue life at 88% and 90% stress levels is huge, more fatigue tests will be conducted at 89% of the ultimate load and other stress levels as needed. Figure 8 shows the failed specimens. Both fiber fracture and delaminations throughout the length of the specimen were observed. Post-impact compression fatigue tests are in progress.

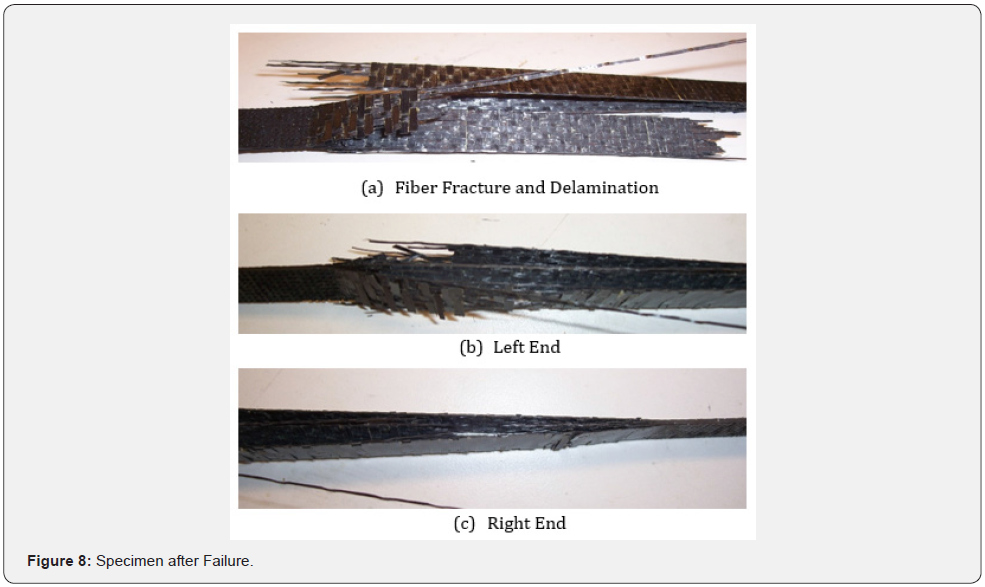

Post-Impact Compression Fatigue Tests
CAI fatigue tests have being conducted on MTM45-1/CF2412 composites manufactured using the OOA process. The laminate construction consists of 8 fabric plies with a stacking sequence of [(+45/-45)/(0/90)]2S. Samples had a thickness of 3.35 mm (0.132 in). The 152.4 mm (6 in.) x 101.6 mm (4 in.) panels were subjected to the 15J of impact energy according to ASTM D7136. The CAI test fixture is edge-loaded between the flat platens as shown in Figure 9. Fatigue behavior of the coupons in sinusoidal compression–compression loadings of 60%, 65%, 70%, 75%, 80%, and 90% of the ultimate strength (224 MPa) or ultimate compressive load of 76.02 kN (17,090 lb) has been used. Initially, panels of 12 fabric plies have been constructed. The ultimate compressive failure load of the specimens was 115.65 kN (26,000 lb). Due to the load cell limits of the available test machines, panels with lower thickness have been chosen. A frequency of 2Hz and a load ratio of R = 10 (R = σmin/ σmax) have been used. The fatigue life of the composites at different load percentiles is given in Table 6. The fatigue curves are shown in Figures 10 & 11. The samples did not fail at 60% of loading even after 700,000 cycles and the tests were stopped.

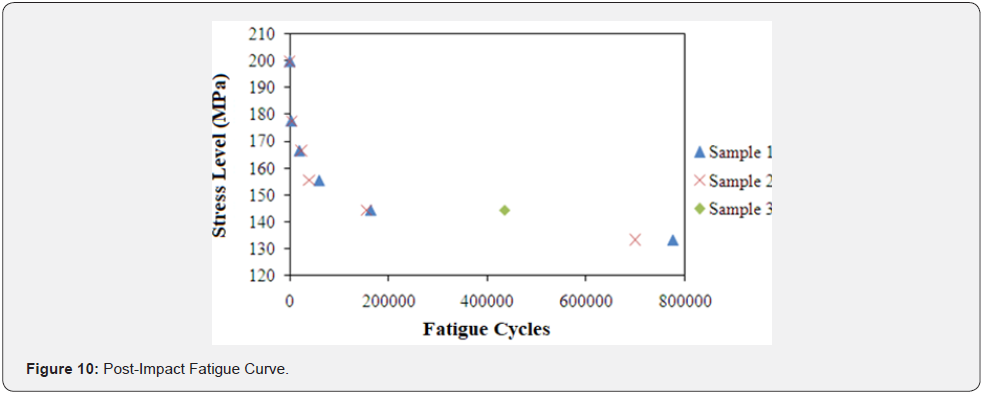

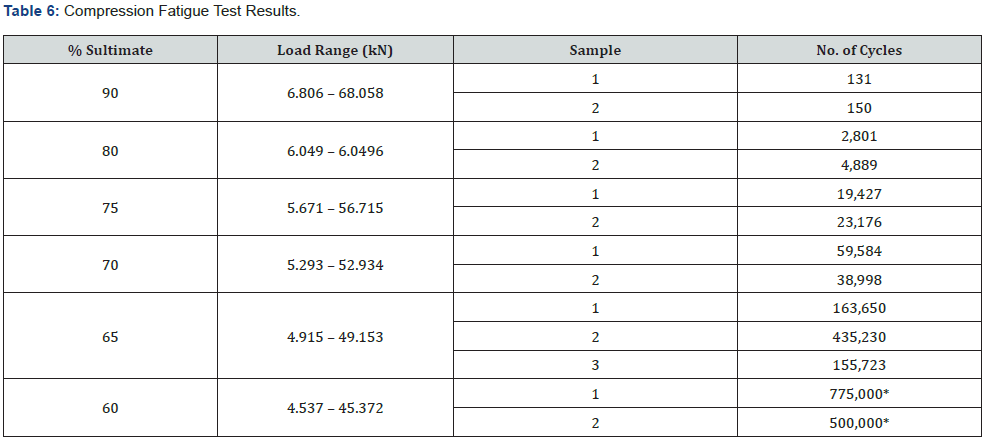
Source: *Did not fail.
Conclusion
A low-cost OOA vacuum-bag-only cure prepreg technology was successfully used to produce high-quality carbon fiber composites with void content less than 0.25 percent. The processing stages that lead to high-quality parts are shown. The fabricated panels were put through tensile, flexure, impact, compression-after-impact, and tensile fatigue tests. The effects of post-impact compression fatigue were studied. The results reveal that the OOA method is capable of producing parts with quality and performance comparable to those produced by the autoclave process at a fraction of the cost.
For more Open Access Journals in Juniper Publishers please click on: https://juniperpublishers.com/
For more articles in Academic Journal of Polymer Science please click on: https://juniperpublishers.com/ajop/index.php
Comments
Post a Comment