Fast Triggered Controllable Electrically Actuated Shape Memory Epoxy: Graphene oxide Nanocomposites
JUNIPER PUBLISHERS- ACADEMIC JOURNAL OF POLYMER SCIENCE
Abstract
Shape memory polymers (SMPs) are the new class of smart fascinating polymer materials with different potential applications. This research work aims to present systematic investigations of electrically actuated shape memory (EASM) properties at different electric voltage of epoxy polymer filled with 0.1 to 0.4 wt. % of Graphene (GO) to develop SMPs nanocomposites. EASM effect and surface morphology functional analysis of composites were observed after EASM characterization by Field Emission-Scanning Electron Microscopy (FE-SEM) and Fourier Transform Infrared Spectroscopy (FT-IR). 0.4 wt. % addition of GO showed highest shape recovery at 180 S, 140 S and 95 S for 40 V, 60 V and 80 V respectively. These results suggest that GO could be promising materials for electrically actuated shape memory applications.
Keywords: Graphene Oxide; Epoxy; Smart Polymer; Shape Memory; Polymer Nanocomposites
Introduction
Shape memory polymers (SMPs) are one of the promising smart materials, which can recover their original shape upon applying an external stimulus, such as heat, light and mechanical force etc [1-3]. Among these SMPs, polymer can recover an original shape in very short period of time from large deformation when subjected to an electrical current. In order to recover the shape of polymer, in case of electrically based SMPs, various types of electrically conductive fillers have been developed, so they can be actuated by passing an electric current. Carbon based nanomaterials such as, CNTs and Graphene have attracted much attention due to their novel properties of electrical conductivity [4-6]. So, development of electrical SMPs filled with carbon nanomaterials can be a way to new research. Most of the research studies found that electrically conductive composites have relatively poor mechanical and thermal properties. By contrast, a new class of thermoset SMPs shows better mechanical and thermal properties than thermoplastic SMPs, and it can be widely used as a functional or a structural material [7-10].These unique characteristics have led SMPs to be used in a myriad of fields, including automobile and aerospace engineering, medical treatment, and many other high-performance applications [9].
Many significant developments have been achieved for SMP composites for which shape recovery actuation can be carried out by electrically resistive heating with respect to maintain the mechanical and thermal properties. However, for the fabrication of electro active SMP composite, almost all previous works were focused on conductive fillers blended into pure polymers with the potential applications of SMPs, but some major limitations still exist and impose major challenges to their broad utilization. Some of the key limitations include low recovery due to low recovery strength, and low recovery speed due to the low thermal conductivity and inertness to electromagnetic stimuli [11]. Among the all conductive materials, GO has tremendous interest in scientific research due to its outstanding electrical responsive shape recovery property. It has two dimensional (2D) and conducting layered one atom-thick planar sheets of sp2-bonded honeycomb structure of carbon atoms. Graphene is a basic building block of graphitic materials having all dimensionalities and used to fabricate/prepare varieties of composite/blend films and polymer nanocomposites. It has extraordinary mechanical [12,13], thermal [14,15], high specific surface, excellent aqueous processability, amphiphilicity and surface functionalizability [7,16,17] and hence extensively investigated for applications in many technological fields [18-24].
It is expected that GO should be able to fulfil its roles as a fixed structure as well as a filler for reinforcement in epoxy-based polymer due to the potential covalent bonds. Larger specific surface area provides a strong connection between GO and polymer. On the other hand, the uniform distribution of GO on surfaces enables good dispersion of GO in the polymer. Even at low volume fractions, the vast interfacial areas created by welldispersed GO can affect the behavior of the surrounding polymer matrix and create a co-continuous network to fundamentally change the physical properties of the polymer matrix [25-27]. In this research article benefits to the electrical actuated shape memory properties of the developed conductive SMP composites were experimentally demonstrated. The improved conductivity also permitted electrical actuation of conductive SMP composites in a low electric power. Epoxy resin was selected as the matrix and GO was as one type of conductive filler for the investigated SMP composites due to their superb electrical, mechanical, and thermal properties; and expected to significantly improve the overall performance of the composite material.
Experimental
Ant
Commercially available Hand Layup Epoxy matrix and hardener, grade name Lapox L-12 and K-6 were procured from polymer division of Atul Limited, Mumbai, India. GO sheets with average 50 nm in thickness and up to the certain μm in length,were synthesized by modified Hummers method which has already been reported in our previous research works [28,29]. Solvents like acetone, ethanol and toluene for experimental and washing purpose were procured from Sigma Aldrich, Mumbai, India. To fabricate the nanocomposites, the epoxy/GO mixture was first prepared with different wt. % (0.1 to 0.4 wt. %) loadings. To lower the viscosity of the epoxy resin, and thus to obtain a good dispersion of modified GO within the matrix, the epoxy resin was diluted by acetone in a ratio of 100:30 and stirred simultaneously with heating up to 60 °C for 12 h for slow solvent evaporation. An additional 12 h of heating under vacuum conditions ensured the complete acetone removal. The hardener was added to the dispersions at a weight ratio of 100:6 and mixed for 5 min. The resulting mixtures were poured into a Teflon mold. In order to characterize and testing, the desired specified samples were cut from the sheets. Rectangular samples (100×10×3 mm3) were cut from the sheet of GO filled epoxy nanocomposites to investigate electrically actuated shape memory effect [30].
Electro-active shape memory testing
The rectangular samples (100×10×3 mm3) of the nanocomposites were heated to the certain temperature and held for 10 min. After getting certain temperature, the samples were bent into a “U” shape and then quickly dipped into ice water to maintain the “U” shape. Different voltages were applied on both ends of the different samples with crocodile pin and recorded the shape recovery process and recovery time.
Characterization
Topographical surface morphology of GO and GO filled epoxy nanocomposites were determined by using field emission scanning electron microscope (FE-SEM, S-4800 Hitachi, Tokyo, Japan) operated at an accelerating voltage of 30 kV). Functional groups of epoxy and GO filled epoxy were recorded on FT-IR spectrophotometer (FTIR-8000 Spectrophotometer, Shimadzu, Tokyo, Japan). The number of scans per sample was 25 and resolutions of the measurements were 4 cm-1. The recorded wave number range was 500-4500 cm-1. Thermal stability of epoxy and GO filled epoxy polymer was determined by a thermo gravimetric analyzer (TGA-50, Shimadzu, Tokyo, Japan). Approximately 10 mg of the sample was placed in a platinum pan for TGA analysis. The temperature range was kept from room temperature to 600 °C and a heating rate was kept 10 °C/min under nitrogen atmosphere to avoid thermo-oxidative degradation.
Results and Discussion
Electrically activated shape memory analysis
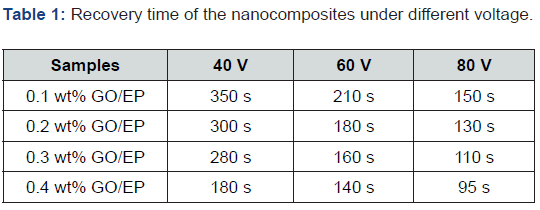
The samples were tested for direct current (DC), interpreted the obtained results, validated and complemented with the references of current research works on enhanced properties achieved with the use of GO. The results are illustrated in Figures 1-5 and Figures S1-S10. A comparable magnitude of improvement in shape memory properties with the different loading and different current are discussed. With the chosen wt. % of GO, the electrically conductive shape memory of the nanocomposite was rapidly improved by increasing the loading of GO and current (V) up to the 0.4 wt. % at 80 V respectively. Figures 1 and S1, S2 show the electroactive shape recovery behavior for the virgin epoxy polymer. The original shape of the sample was almost same; there was no change observed when an electric field of 40 V and above i.e. 60 and 80 V was applied. In the beginning at 0.1 wt. % (Figures 2 and S3, S4), a change was observed in shape memory of nanocomposites in 350 S at 40 V which further increased the shape memory properties in less time i.e. 210 S and 150 S for 60 V and 80 V respectively (Table 1). Figures 3-5 show the electro active shape recovery behavior for the epoxy containing 0.2, 0.3 and 0.4 wt. % GO. The original shape of the nanocomposites was almost completely recovered in 0.2, 0.3 and 0.4 wt. % for all the electric fields. From the figure 100(±2) % recovery were observed in 0.2- 0.4 wt. % loading of GO. The comparison of shape recovery was 0.2 to 0.4 wt. % loading with different electric fields and degree of shape recovery are shown in Figures S5-S10 and also mention in Tables 1-4. It is observed from the tables that the rate of shape recovery was strongly dependent on the magnitude of the applied voltage and the GO wt. % content in the polymer (at 0.4 wt. % loading shape recovery time was 95 S for 80V) [31]. But at lower loading (0.2 wt. %) from our previous research study [30] found that it provides better water thermal sensitive shape memory properties. The GO filled composites could be heated more rapidly to a higher temperature with higher voltage application (80 V) in comparison that of the lower voltage (40 and 60 V) because of their higher conductivity.

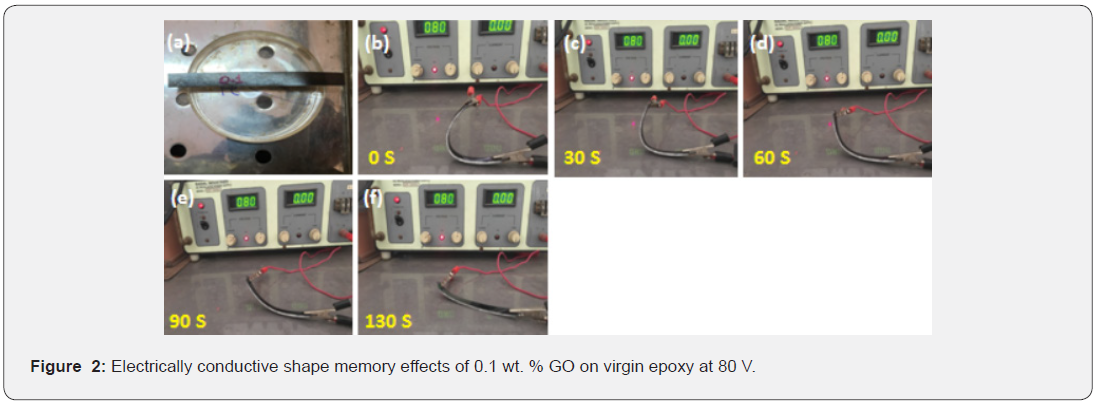
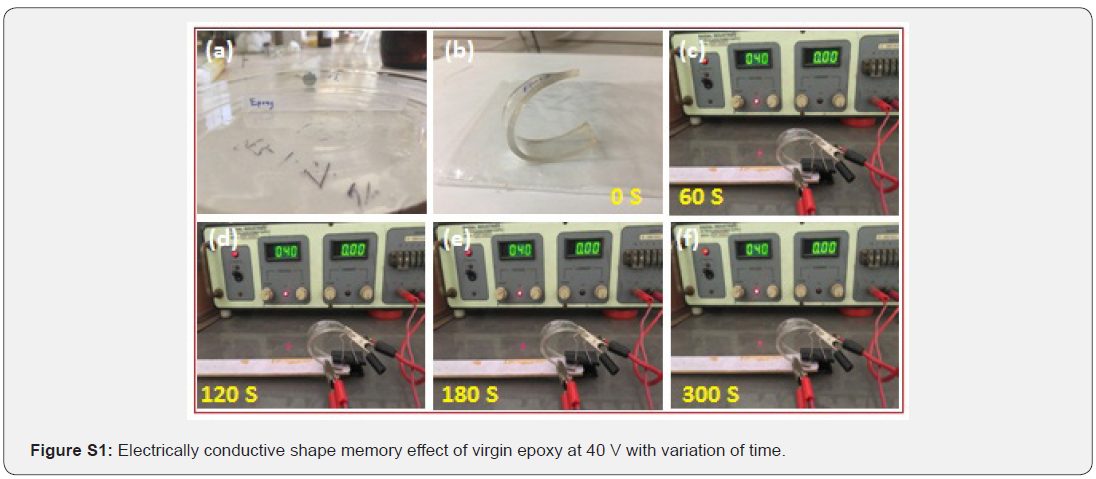
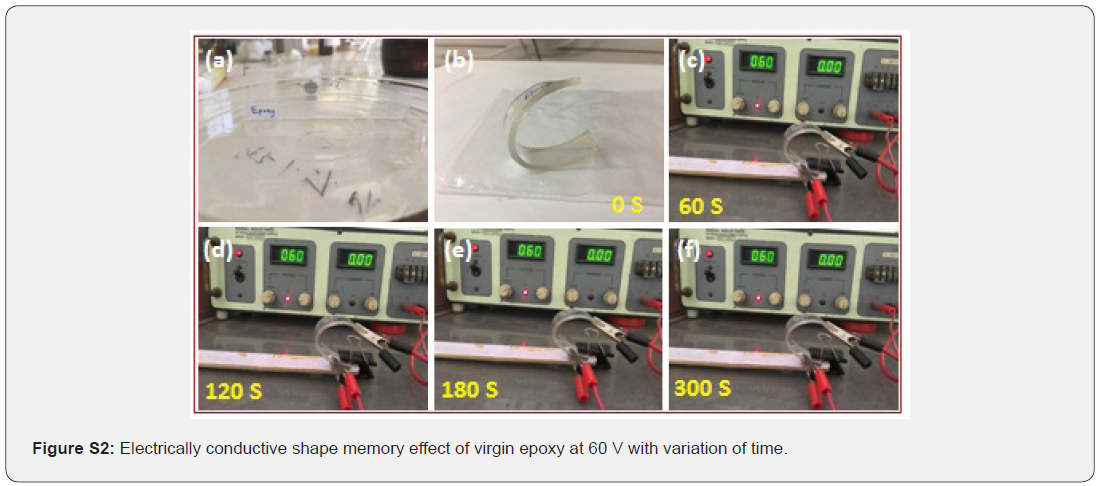
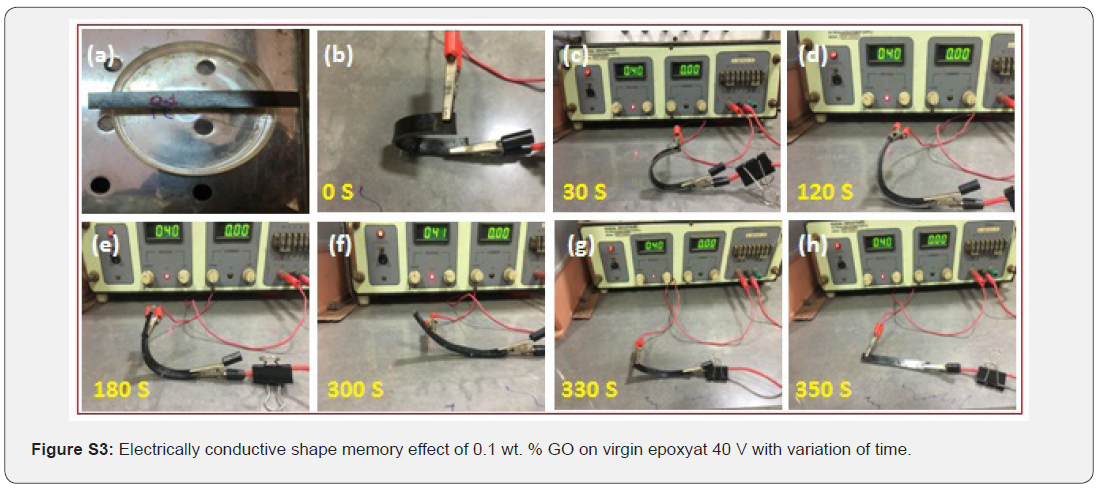
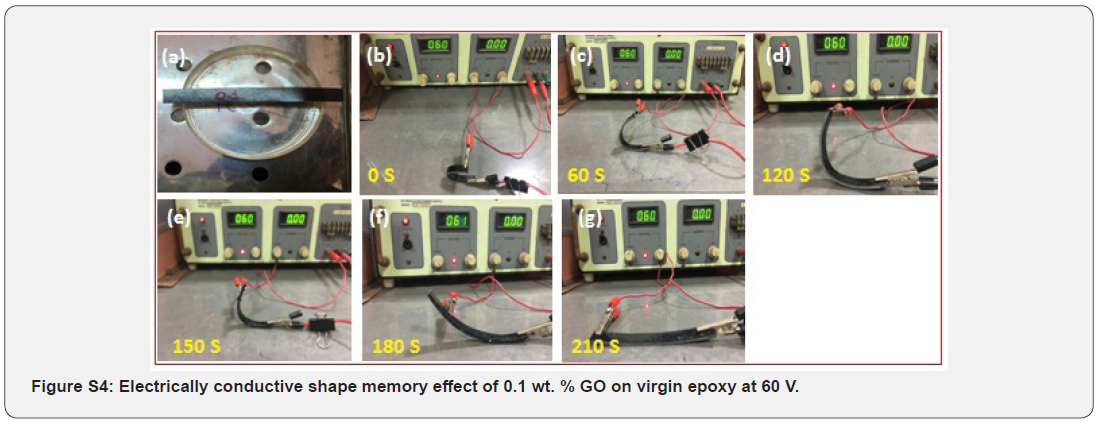
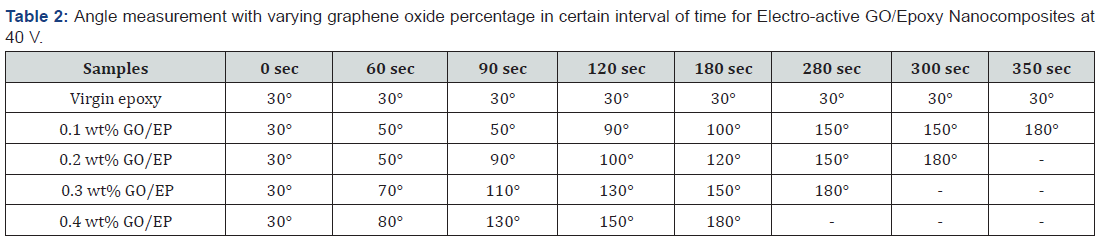
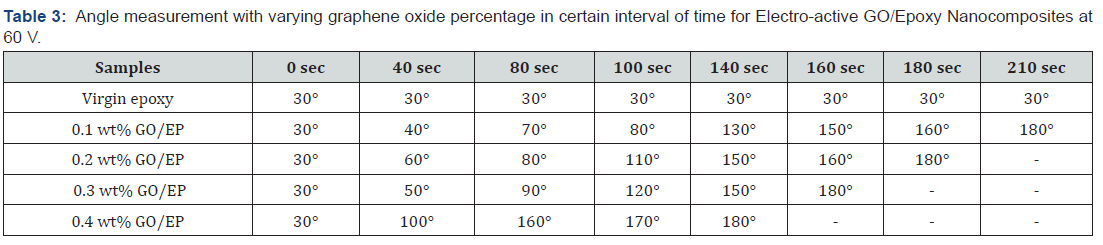
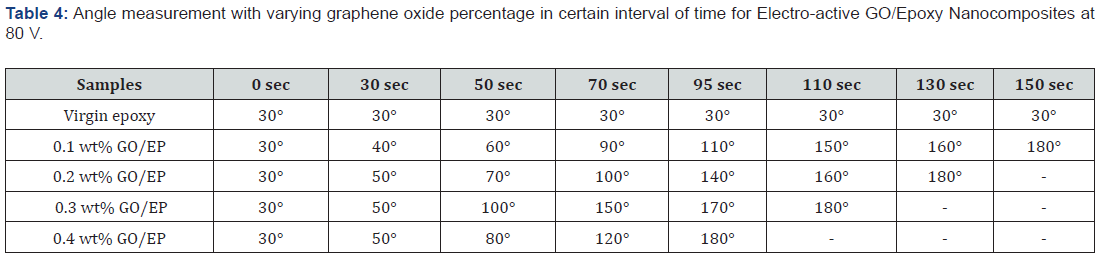
Morphological and functional analysis of composites
Morphological Study and functional analysis are rather important in order to explain and interpretate the reason behind electrically conductive shape memory properties. Cross sectional FE-SEM images of GO filled epoxy (0.1 to 0.4 wt. % loading) after electrically conductive shape memory testing at 80 V are shown in Figure 6. It shows different morphological changes at different wt. % loadings. At the initial stages of 0.1 and 0.2 wt. % loading (Figure 6 a and b), the GO sheets are properly embalmedand exfoliated with epoxy matrix. But at 0.3 and 0.4 wt. % loading (Figure 6 c and d), there is availability of higher amount of GO with distributed and aggregated nature towards the whole polymer matrix and it provides a higher and fast electrical conductivity. So, the electrical actuation in the case of 0.4 wt.% loading is higher than lower wt. % loadings (0.1 and 0.2. wt. %). But in our earlier research work of GO filled epoxy and their thermally water sensitive shape memory behavior, it was found that GO creates better stacking galleries in epoxy at lower loading (0.2 wt. %) and that have generate a specific and rapidly thermally water sensitive properties as compared to 0.3 and 0.4 wt. % loading [30].
In the case of functional analysis, FTIR was used to characterize and differentiate between composites with electrically conductive shape memory polymer composites. From the FTIR spectra (Figure 7), no much consistent difference was observed in GO filled epoxy at 0.1 to 0.4 wt. % loading; and suggested that only physical changes were found in composites. Figure 7(d) shows the strong and broad peak at 3360 cm-1 in 0.3 wt. % indicating the presence of surface O–H stretching due to the vibrations of the H-O-H groups of water. The other peaks correspond to oxygen functional groups, such as carboxyl (C=O) stretching of COOH groups (1725 cm-1), aromatic (C=C) stretching (1615 cm-1), epoxy (C–O) group stretching (1218 cm-1) and alkoxy (C–OH) group stretching vibrations (1043 cm-1) indicate the presence of GO.
Thermal properties of composites
Figure 8 shows TGA curves of the neat epoxy and its composites with 0.1 to 0.4 wt. % loading. As can be seen from the curves, all the samples begin to decompose at 370-390 °C after electrical active shape memory testing and degrade almost completely below 445 °C due to the pyrolysis of polymer mainchains. It should be noted that the thermal stabilization that is onset thermal degradation temperature (don) of the composites was observed to be increased with increase in GO loading (Figure 8 and Table 5). The higher don degradation temperature was recorded as 391 °C and offset thermal degradation temperature (doff) as 447 °C for 0.3 wt. % loadings (Table 5). In case of thermal properties of the GO filled epoxy, it is observed that don and doff temperature were increased with increase in weight loss before any actuation [18]. But after the electrical actuations, don and doff were observed to be increased with increase in GO loading with decrease in weight loss as compared to characterization of thermal properties before electrical actuation. This happened when electric current passes through the higher amount of GO, there is occurrence of electrical resistive heating in the GO. The resistive heating is transferred from the GO to the epoxy matrix resulting from the temperature difference [32].
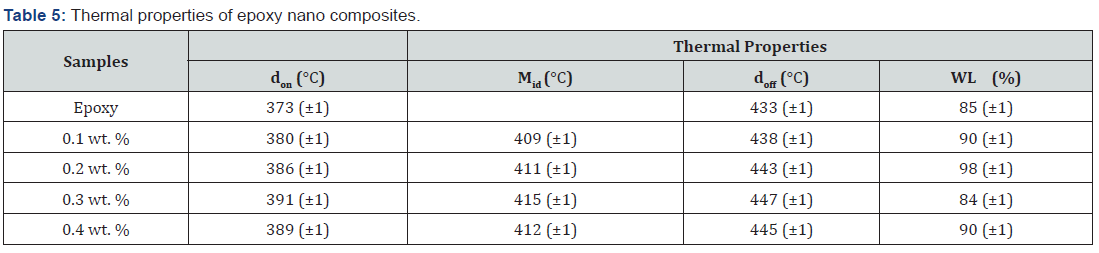
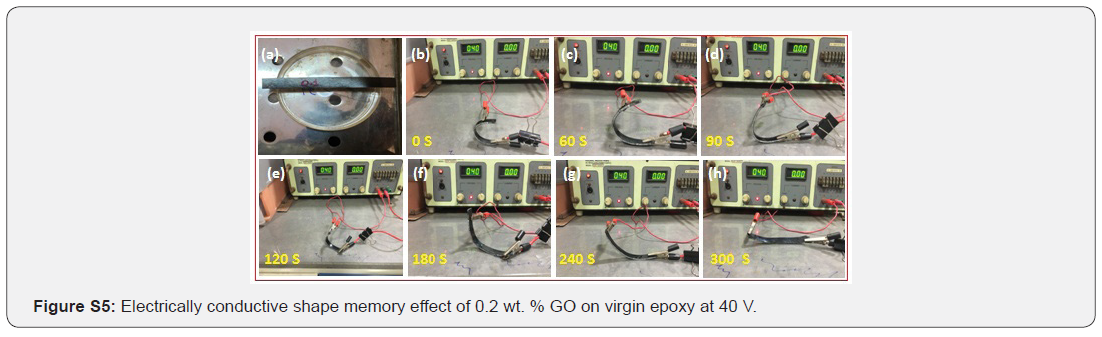
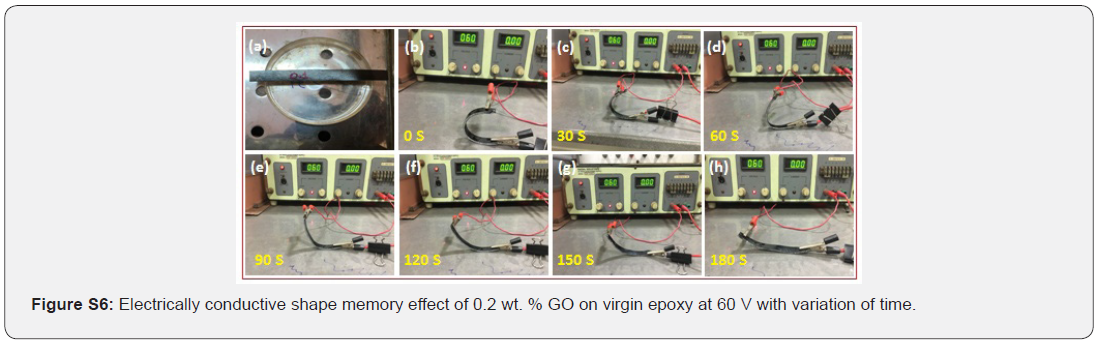
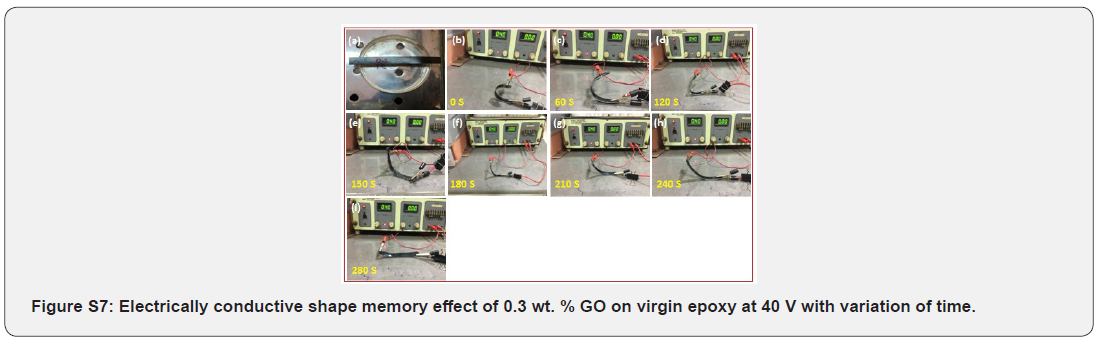
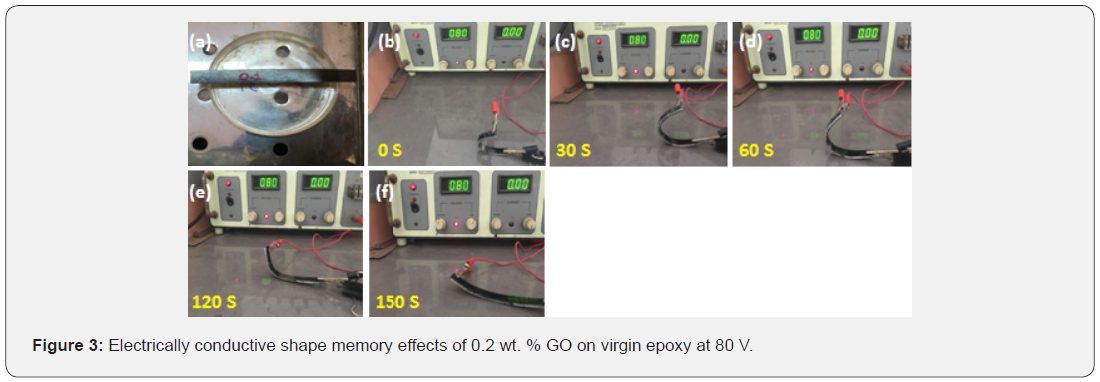
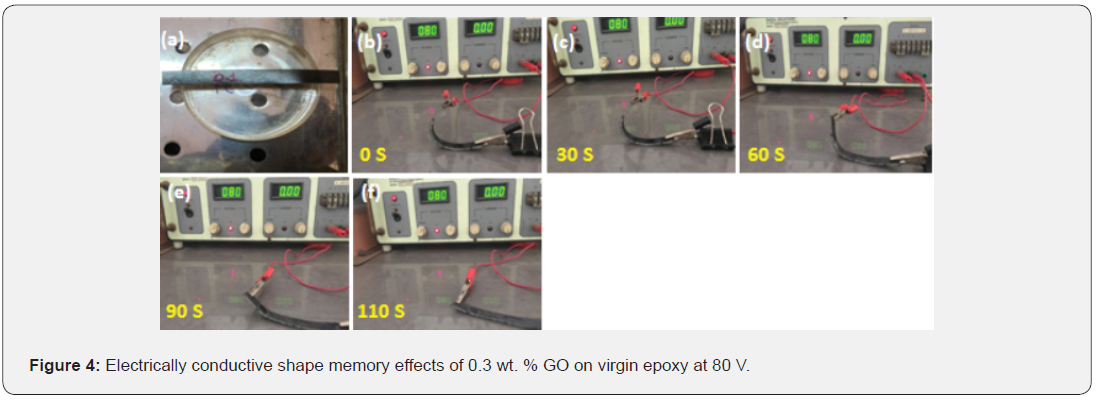
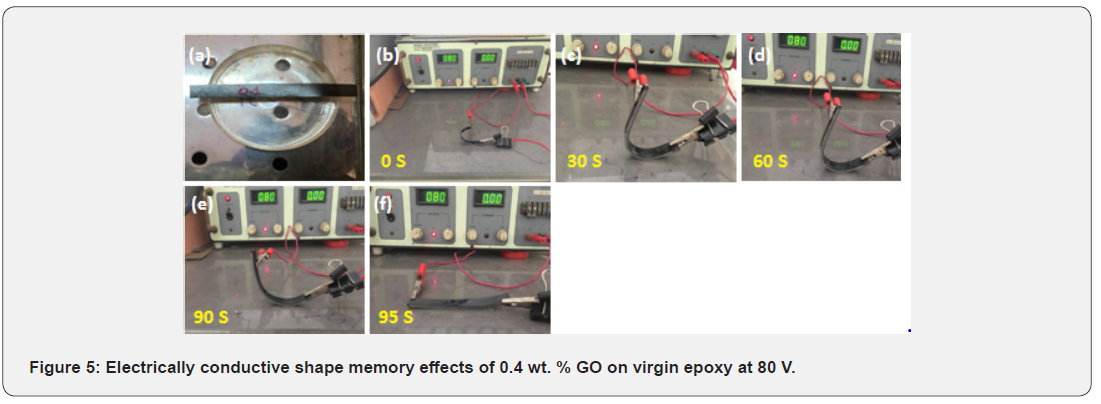
Conclusion
This research work presented a new approach to significantly improve the electrical actuated epoxy shape memory of epoxy nanocomposites embedded with GO. With the varying amount of GO, the electrically actuated shape memory property increased with increase in both GO wt. % loading and electric voltage.The actual electrically actuated shape memory property at 0.4 wt. % loading is 180 S, 140 S and 95 S with 40 V, 60 V and 80 V as compared to 0.3 wt. % loading (280 S, 160 S and 110 S for 40 V, 60 V and 80 V respectively).The demonstrated experimental shape memory results showed that GO filled epoxy composites have potential electrical conductivity.Which will be beneficial for their applications in a shape recovery forces output.
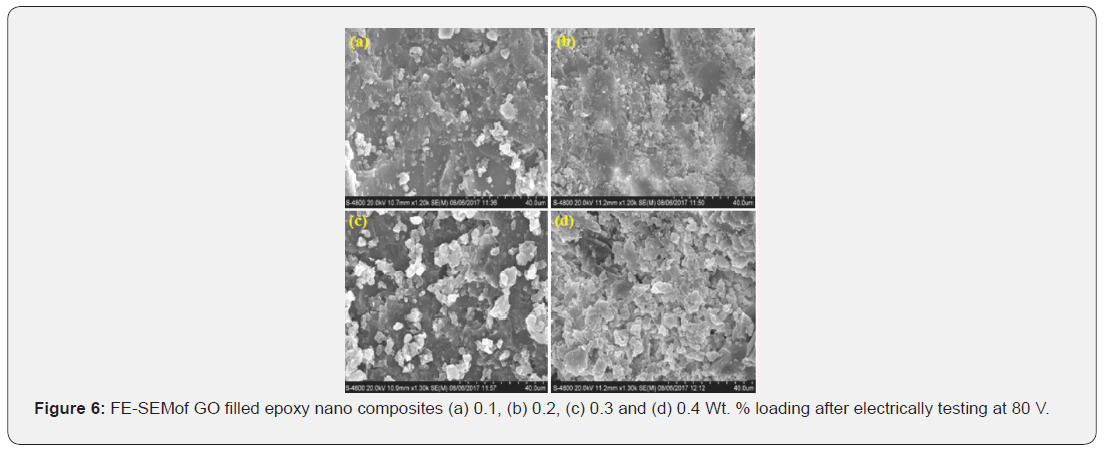
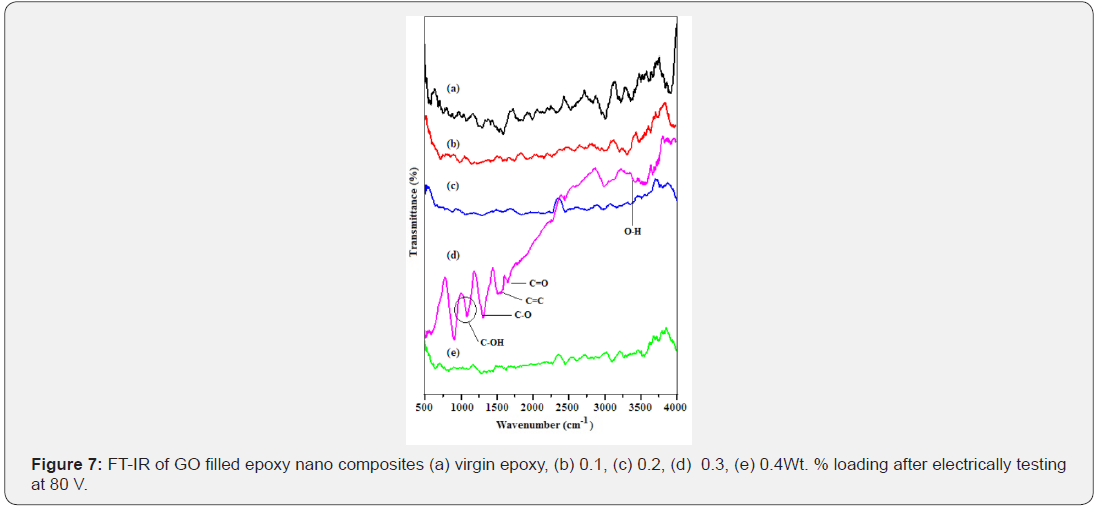
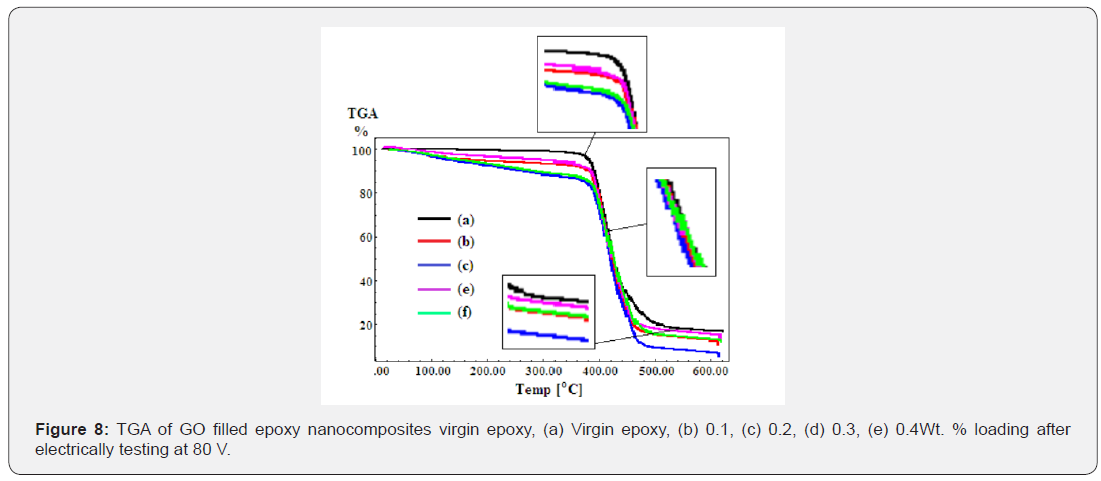
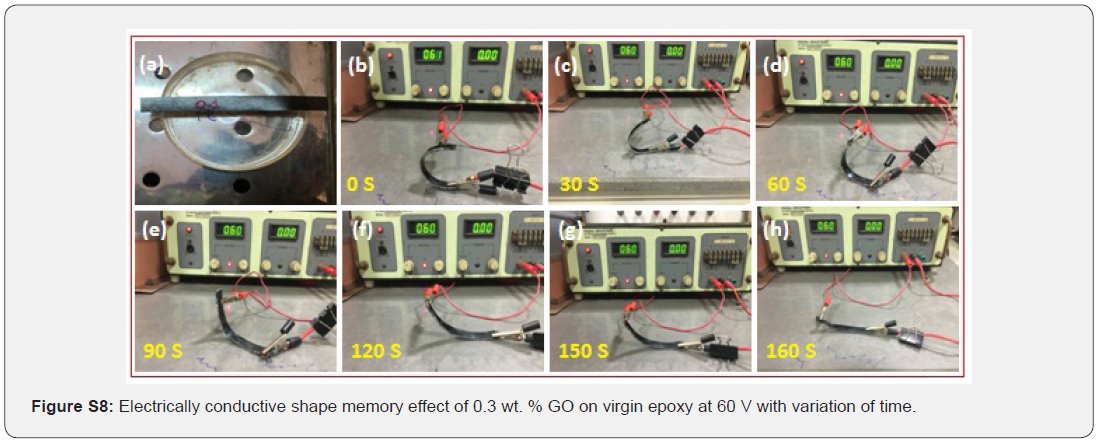
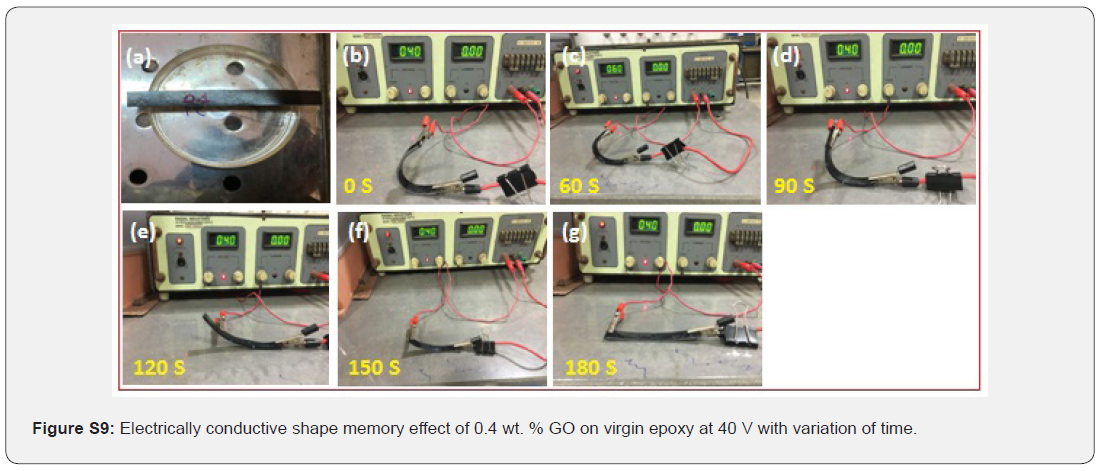
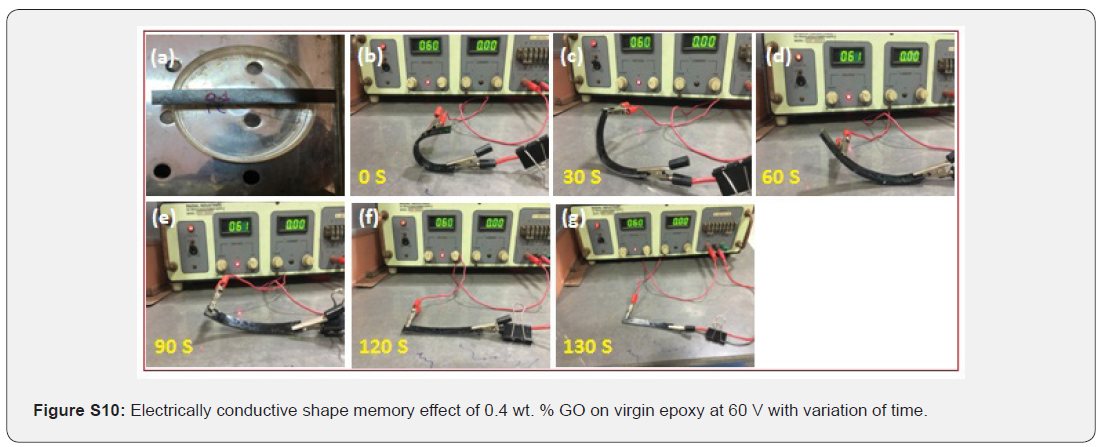
For more Open Access Journals in Juniper Publishers please click on: https://juniperpublishers.com/
For more articles in Academic Journal of Polymer Science please click on: https://juniperpublishers.com/ajop/index.php
For more about Juniper Publishers Please click on: https://juniperpublishersblog.wordpress.com/
Comments
Post a Comment