Synthesis of Chitosan-Co-Lactic Acid Nanoparticles and their Potential as a Carrier of Anticancer Drug
JUNIPER PUBLISHERS- ACADEMIC JOURNAL OF POLYMER SCIENCE
Abstract
This study aims to design some medicinally important biopolymer based Nano carriers. The study undertakes synthesis, characterization of Chitosan-co-lactic acid nanoparticles and optimization of swelling ratio. The Chitosan-co-lactic acid nanoparticles were prepared by the shaking of lactic acid emulsion in Chitosan solution by emulsion crosslinking method and characterised by transmission electron microscope (TEM) analysis (size), and Fourier transform infrared spectra (FTIR) (structural) and SEM (morphology). The particles were dispersed in a phosphate buffer saline (PBS) and the swelling response of these nanoparticles was studied as a function of chemical composition, pH and temperature of swelling medium. To determine the encapsulation efficiency, a centrifugation method was applied. Also, the assessment of biocompatibility has been made on the basis of two in-vitro test which are BSA adsorption test and haemolysis assay. Our result revealed that equilibrium swelling was influenced by the composition of nanoparticles and the swelling medium. These observations and assessment indicate that these nanoparticles have good capability for use in drug delivery and suitable for biomedical application.
Keywords:Chitosan; Nanoparticles; Swelling Behaviour; Drug Delivery Devices; Lactic Acid
Introduction
Cancer is a disease which refers to a group of tumour which is formed by an excessive growth of abnormal cells [1]. Cancer is characterized by uncontrolled cell growth, and the potential to get larger into neighbouring tissues and to metastasize. Surgery may not be always more effective due to the involvement of the tumour with neighbouring tissues [2]. In such conditions, Chemotherapy (a destructive agent) is a conventional approach with the major potential to cure localized and metastasized cancers. Chemotherapy is a general term used to describe using several drugs that are destructive to malignant cells and tissues [3]. Anticancer drugs are effective against a wide range of tumours, formulated to treat wide range of tumours but its clinical applications are inhibited due to its poor solubility [4]. The main drawback of these drugs is that they not have enough of selectivity towards tumour cells. Because of the weak selectivity, they kill both normal and cancer cells and result in toxicity to normal tissues [5,6]. The target site-specific delivery can be attained by a drug delivery system. Drug delivery systems can reduce the side effects of anticancer drugs via encapsulation methods, which decrease the scrape of toxic drugs, protect the chemotherapeutic drug prior to attaining the target cells. Encapsulation is one of the effective procedures, as this not only enhances water solubility of the drug but also reduces gastrointestinal impatience and provides a long-lasting release rate [7,8]. The purpose of chemotherapy is the sustained, controlled, and targeted delivery of anticancer drugs for a long period which can be achieved by polymeric nanoparticles [9].
A drug delivery system is generally associated with particulate carriers, such as polymeric micelles [10], emulsions, liposomes [11] and nanoparticles (NPs) [12], which are devised to localize drugs at the target sites. The nanoparticles are submicron-sized, colloidal particles that carry drugs to the target or release drugs in a controlled manner in the body. Controlled drug delivery offers advantages like reduced dosing frequency, better therapeutic control, reduced side effects, and, consequently, better patient compliance. After preparation, nanoparticles are usually dispersed in liquid [13-15]. Recently, polymer nanoparticles have attracted significant interest in chemistry, pharmaceutics and biomaterial science for biomedical application and controlled release [16]. Both synthetic and natural polymers were studied with the purpose of making nanoparticles [17]. Nanoscale drug delivery systems are made from biocompatible and biodegradable material constitute an extend to drug delivery and tumor targeting [18].
However, among the variety of polymers that were used for drug-loaded nanoparticles, chitosan has received great attention in both the medical and pharmaceutical fields [19]. Chitosan, a biodegradable and biocompatible polymer, is a modified natural carbohydrate and the second most abundant polysaccharide in nature [20]. It can be synthesized by the partial N-deacetylation of chitin, chitosan has important applications in various areas and has been the topic of much research in recent years. Since the amine group −NH2 can be protonated to NH3+, which may provide chitosan with a favourable gel forming property, it has been all inclusive examined in the medical industry for its potential in the development of pH-sensitive drug release systems [21]. It consists of repeating units of glucosamine and N-acetyl-glucosamine, the proportions of which determine the degree of deacetylation of the polymer. Chitosan is available in a wide range of molecular weights and deacetylation degrees. Due to its characteristics, chitosan has attained increasing attention in the pharmaceutical field [22]. As one of the well-recognized organic acids, lactic acid has established its potential for use in several biomedical applications due to its antibacterial and excellent biocompatible properties with low toxicity and bio-absorbability in vivo [23, 24]. It has been widely used in biomedical applications, such as sustained drug delivery systems, implants for orthopaedic devices and absorbable fibres [25]. Chitosan and Lactic acid have highly pH-dependent swelling properties, which could be used to control the release of drugs and may be used to treat cancers.
Chemotherapy is a conventional approach with the major potential to cure localized and metastasized cancers [26], in which drugs that are commonly used in cancer therapy are infused through the blood vessels [27] (Figure 1).
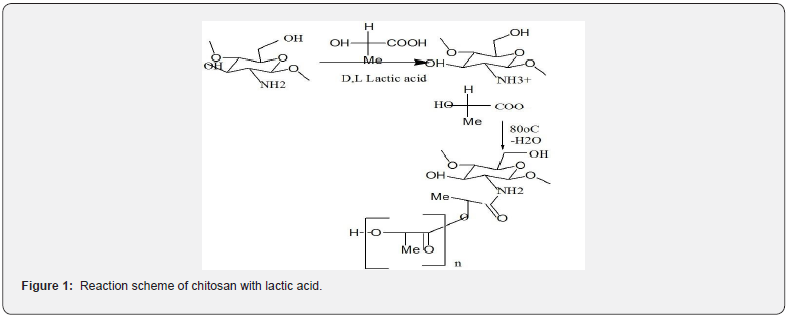
The intravenous injections cause drug decomposition in the blood after an initial rapid increase in concentration [28]. Drug delivery system can reduce these inefficiencies via encapsulation methods, which decrease the lesions of drugs. Even though anticancer drug proves to be remarkably nontoxic and has promising medicinal properties, its relevance in anticancer therapies is inadequate owing to its low aqueous solubility and poor bioavailability. These drug formulations are higher to conventional medicines with deference to control release, targeted delivery and therapeutic impact. Polymeric nanoparticles have concerned noteworthy consideration in the study of drug delivery systems as they offer a means for localized or targeted delivery systems of a drug to specific tissue/organ sites of interest with an optimal release rate [29]. Polymeric nanoparticles act as nanocarriers with many advantages, such as low toxicity and high stability. In this study, the Chitosan-co-lactic acid nanoparticles were prepared by emulsion crosslinking method. The Synthesized nanoparticles were characterized by Fourier Transform Infrared (structural), Scanning electron microscopy (morphology of nanoparticle surface), Transmission electron microscopy (size range) and their water imbibition capacity has been evaluated under varying experimental conditions. The present work aims to preliminary study will be determined for nanoparticles drug loading capacity, encapsulation efficiency and in vitro bioavailability of nanoparticles using UV-VIS spectrophotometer.
Materials and Methods
Materials
Chitosan was obtained from Hi Media Pvt. Ltd, (Mumbai, India) and Lactic acid, paraffin oil and Glutaraldehyde were purchased from Loba Chemie (Mumbai, India). By using disodium hydrogen orthophosphate, Phosphate buffer saline (PBS) was prepared. Throughout the experiments all other chemicals were of analytical grade and used as received without further purification.
Methods
A. Preparation of Chitosan-co-lactic acid nanoparticles
Chitosan – Co- Lactic acid nanoparticles were prepared using an emulsion crosslinking technique with minor alteration in protocol [30]. Briefly, aqueous phase of chitosan was prepared by dissolving the appropriate amount of chitosan in acetic acid solution (2.5%) and then poured in paraffin oil i.e. oil phase with vigorous shaking for 30 min (shaking speed 300 rpm) at the same time emulsion of lactic acid was added to the mixture with uniform shaking. After some time, emulsion of gluteraldehyde was added to the mixture with uniform shaking. The reaction mixture was kept for predetermined time period to attain crosslinking reaction. After that synthesized nanoparticles were separated from the medium by washing thoroughly with toluene, acetone and dried. The dried and powder nanoparticles were kept in air tight polythene bags and were subjected to further analysis. The advantage of this method was credited to its mild conditions achieved without applying harmful organic solvent, heat or vigorous agitation that are damaging to sensitive proteins.
Characterization of Chitosan-Co-Lactic Acid Nanoparticles
Nanoparticles were characterized at two levels which one is physiochemical and the second one is biopharmaceutical characterization.
Physiochemical characterization – Under these level of characterization nanoparticles was characterized by the following methods:
FTIR spectral analysis: FTIR was used to characterize the structure of chitosan-co- lactic acid nanoparticles. The IR spectra of chitosan-co- lactic acid nanoparticles were recorded by a Shimadzu, Japan. Model- 8400S FTIR spectrophotometer and the samples were prepared by KBr pellet technique.
SEM analysis: The morphology of chitosan-co-lactic acid nanoparticle was observed using a scanning electron microscope (SEM) (HITACHI S-3700N) and the samples were coated by gold.
TEM analysis: Transmission electron microscope (TEM) was used to examine the size of nanoparticles using JEOL (JEM-2100) Japan with an acceleration voltage of 200 kV
Particle Size analysis: The distribution of Chitosan-co- lactic acid nanoparticles were characterized by Malvern instrument Ltd. for mean particle size (mean intensity weighted diameter, Zaverage) and poly dispersity index (width of the size distribution, PDI).
Biopharmaceutical Characterization
The swelling behaviour of Nanoparticles influence their usability as a biomaterial in medicine pharmaceutical.
Swelling Ratio
The mechanism of water diffusion into the nanoparticles is important for assessing the suitability of nanoparticles as drug delivery, as the amount of drug released is dependent on the rate and transport mechanism of water diffusing into polymer matrix. The swelling response of Chitosan-co- lactic acid nanoparticles was determined by an accepted gravimetric method [31]. In a typical experiment appropriate amount of nanoparticles were dispersed in an applicable volume of Phosphate Buffer Saline (10 ml) until equilibrium was reached. The representation of polymer relaxation during water sorption has been shown in Figure 2. The swelling ratio of nanoparticles was calculated from the following equation-
Swelling ratio= Wt / W0
Where, Wt is the weight of nanoparticles (time t)
W0 is the weight of nanoparticles (time 0)
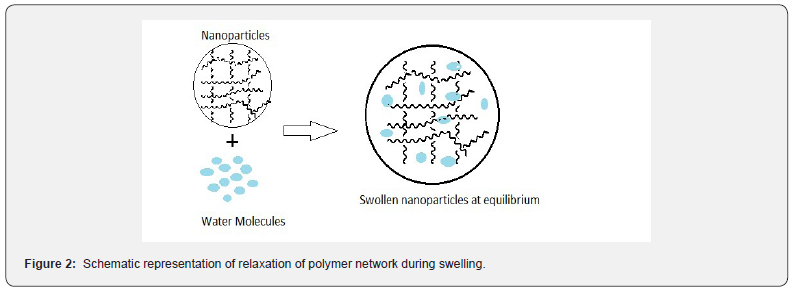
Drug Loading and Encapsulation Efficiency
Drug loading capacity and encapsulation efficiency was dogged by centrifugation method with slight modification in the protocol [32]. The mixture of nanoparticles in drug solution was centrifuged at 10,000 rpm for 2 hr and the amount of free drug in the supernatant was determined by UV spectrophotometer. The drug loading and drug entrapment efficiency were defined by the following equations respectively: % loading = Wd – Wo/ Wo (Wd – weight of drug loaded NPs, Wo – weight of dry NPs)
% EE = (A – B/ A )* 100
Where A - Total amount of drug loaded B- free drug in supernatant
Invitro Cytotoxicity
The MTT assay was performed to measure the metabolic activity of cells to reduce yellow colored tetrazolium salt 3-(4,5- Dimethyl thiazol- 2-yl)-2,5- diphenyltetrazolium bromide to purple colored formazan. Prior to extraction test materials was sterilized with acetone under aseptic conditions. Extract was prepared by incubating test material chitosan- lactic acid in physiological saline at 37+1O C for 72 + 2h at an extraction ratio of 0.2g/ml. The extract was mixed with MEM2X medium to get 50% extract. This was further diluted with culture medium to 25%, 12.5% and 6.25%. Physiological saline without test material processed similar to test material extract was considered as reagent control. Cells cultured in normal medium was considered as cell control. Equal volume (100μl) of various dilution of test samples, extract of negative control, cell control and Positive control were placed on sub confluent monolayer of L-929 cells. After incubation of cells with various concentration of test sample and controls at 37+1 C for 24+2h, extract and control medium were replaced with 50μl MTT solution, wrapped with aluminum foil and were incubated at 37+2 C for 2h. After discarding the MTT solution, 100 μl of Isopropanol was added to all wells and swayed the plates. The color developed was quantified by measuring absorbance at 570 nm using a spectrophotometer. The data obtained for test samples were compared with cell control.
Evaluation of In Vitro Blood Compatibility
Protein Adsorption
In the present investigation compatibility of nanoparticles has been judged by monitoring the amount of protein adsorbed by the nanoparticles. When biomaterials come in contact with biological fluid such as blood, protein within millisecond begin to adhere to the surface through a process known as protein adsorption. Protein adsorption is the first process that occurs after implantation of a biomaterial in the human body [33,34]. The foremost event occurring at the interface of the blood- material contact is the adsorption of plasma proteins (bovine serum albumin, fibrinogen, etc.) which subsequently influences the adhesion of leukocytes, macrophages or platelets and ultimately leads to fibrous encapsulation. Thus, the adsorption of proteins could be one of the determinants of biocompatible nature of the material [35]. The adsorption of proteins was studied as below: A known volume of protein solution of definite concentration was mildly shaken with the known quantity of nanoparticles for a definite time period and the remaining concentration of protein was monitored in the supernatant solution spetrophotometrically. The amount of the adsorbed protein was calculated with the help of the following mass balance equation. Adsorbed amount (mg m-2) = (Co-Ca) V / A
Where, Co and Ca are the concentrations of protein solution (mg per mL) before and after adsorption, respectively. V is the volume of the protein solution and A is the surface area of the adsorbent.
Haemolysis experiments were performed on the surfaces of the prepared nanoparticles as reported elsewhere [36]. In this experiment, dry nanoparticles were equilibrated in normal saline water (0.9% NaCl solution) for 24 h at room temperature and human blood was added. After 20 min, 20 mL of saline was added to stop haemolysis and the sample is incubated for 60 min at 37 0C. Positive and negative controls were obtained by adding 0.025 mL of human ACD blood and saline solution, respectively to 2.0 mL of distilled water. Incubated samples were centrifuged for 45 min, the supernatant was taken out and its absorbance was recorded on a spectrophotometer at 545 nm. The percent haemolysis may be calculated using the following relationship.
% Hemolysis = A test sample – A (-) control / A (+) control – A (-) control Where A = Absorbance.
Results and Discussion
Synthesis of Chitosan-co- lactic acid Nanoparticles
The Chitosan-Co-lactic acid nanoparticles were synthesized by emulsion crosslinking method. Chitosan Co-lactic acid nanoparticles were formed instantaneously due to the protonated amino groups of chitosan and carboxyl groups of lactic acid electrostatically interact. The representation of nanoparticles synthesis polymer has been shown in Figure 3.
FTIR Spectrum
FTIR spectral analysis is a common method for investigating the intermolecular and intramolecular interaction in the nanoparticles. The observed spectra of chitosan, chitosan-colactic acid are shown in (Figures 4a & 4b). The peak assignment of chitosan was as follows (cm−1): 3468 cm-1 (O-H stretch overlapped with N-H stretch), 2922 cm-1 (C-H stretch), 1699 cm-1 (amide I band, C=O stretch of acetyl group), 1597 cm-1 (the bending vibrations of the N–H), 902 cm-1 and 1157 cm-1 (saccharide structure), 1367 cm-1 (CH3 symmetrical deformation). After the conjugation reaction, the absorption band of C-H stretch at 2922 cm-1 observably shifted to 2955 cm-1 and the absorption band of C-N stretch at 2141 cm-1 shifted to 2154 cm-1, the absorption band of O-H stretch overlapped with N-H stretch at 3468 cm-1 and shifted to 3458 cm-1. All these differences pointed out that carboxyl group of lactic acid was successfully conjugated to the amino group of chitosan. Moreover, the crosslinking of chitosan & lactic acid by gluteraldehyde also occurs via electrostatic forces.
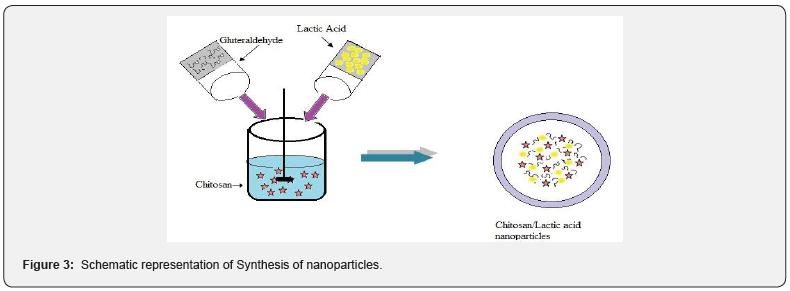
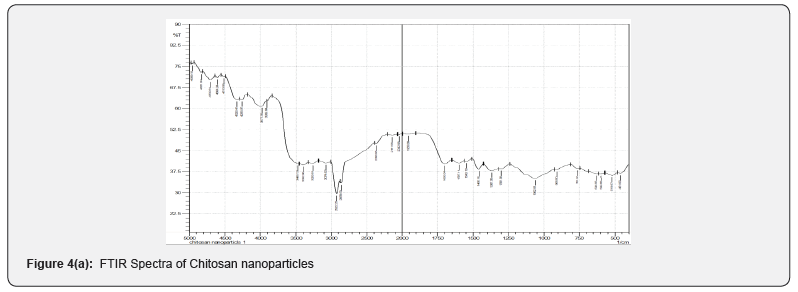
SEM
The SEM image of nanoparticles is shown in Figure 5. A Figure 5 is clearly suggests that the chitosan-lactic acid nanoparticles has non smooth morphology, fracture surface and dispersed with aggregates.
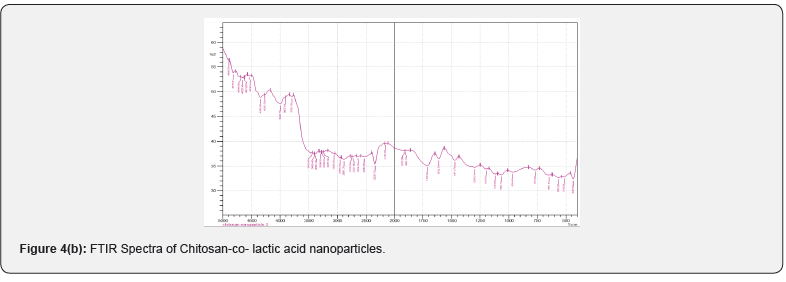
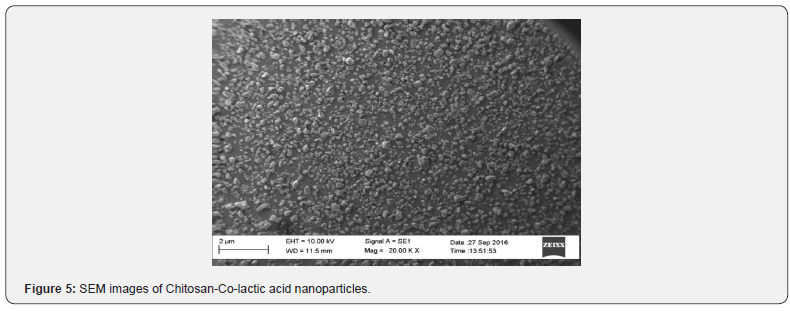
TEM
The nanoparticles are eventually convenient for any pharmaceutical purpose or not, is decided by one of important factor i.e. the size of nanoparticles. It has been reported that the smaller the size of nanoparticles is, the larger their circulation time in blood vessel. In this study, size of nanoparticles which is characterized by TEM is shown in Figure 6, which clearly shows that an average size range of about 100- 180 nm with nearly spherical shaped nanoparticles were synthesized.
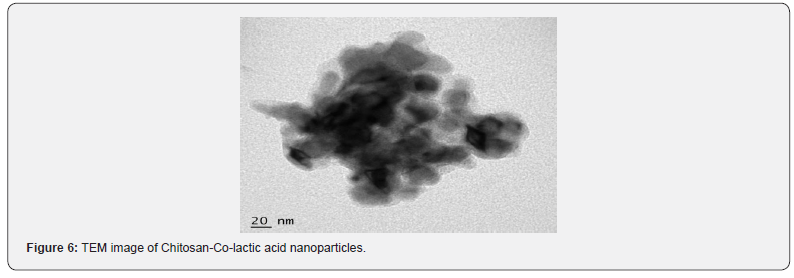
Particle Size analysis
The distribution of particles was evaluated by the polydispersity index (PDI). When the amounts of PDI varied between 0.01-0.7, the dimensional distribution is narrow & in high values of PDI> 0.7 particle have a wide dimensional distribution. According to the PDI of sample (PDI 1), it could be deducted that the nanoparticles had a wide dimensional distribution with Z (Figure 7). av 334.5d-nm. The colloidal stability of nanoparticles and nanoparticle surface charge was observed by means of zeta potential measurement. The nanoparticles have a susceptibility to accumulate, which may dangerous if those particles are injected intravenously because it leads to precipitation. The value of zeta potential for nanoparticles is -14.4 mV, which indicate that nanoparticles have charged surface.
Effect of Chemical Compositions
The swelling behavior of the nanoparticles are mostly affected by the amount of components, functional groups (hydrophobic and hydrophilic) of the polymer. We have studied in this part the influence of functional groups of the chitosan and lactic acid on the swelling behavior by varying the quantities of their concentrations.
Effect of pH
The effect of variation of pH on the swelling behaviour has been examined by performing water sorption experiments at different pH 2.6, 7.4 and 9.0. The observed results are shown in Figure 8, which reveal that the swelling behaviour at different pH values gets significantly affected. It was observed that the swelling behaviour of nanoparticles was maximum at pH 2.6 and above this pH, it started to decrease, which may be explained as below. Due to low pH, nanoparticles were swelled immediately as a result of osmotic pressure and charge repulsion between the ions present in nanoparticles. The swelling behaviour of nanoparticles was more in acidic medium than the neutral and basic medium. The chitosan has pKa value of about 6.5 due to amino group which leads to a protonation and increased charge density of the nanoparticles may enhance the osmotic pressure inside the particles due to electrostatic repulsion between NH2 +- NH2 + group. This osmotic pressure difference between the internal and external solutions of the particles is balanced by the water sorption. While at higher pH, swelling ratio is decreased due to the deprotonation of the amino units of chitosan. In addition, the hydrophobic sidechain aggregation and hydrogen bonds in the copolymers are much stronger, which will also lead to lower swell ability of the samples. The results revealed that the nanoparticles changed their swelling property when the environmental pH is changed. The water permeability can be converted in response to a change in the environmental pH, it would be a desirable characteristic for a pH sensitive controlled release system with controllable swelling ability (Figure 9).
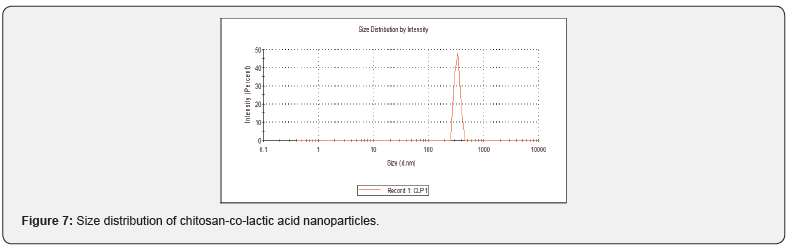
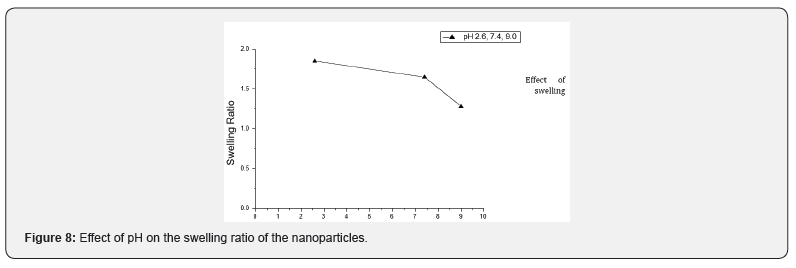
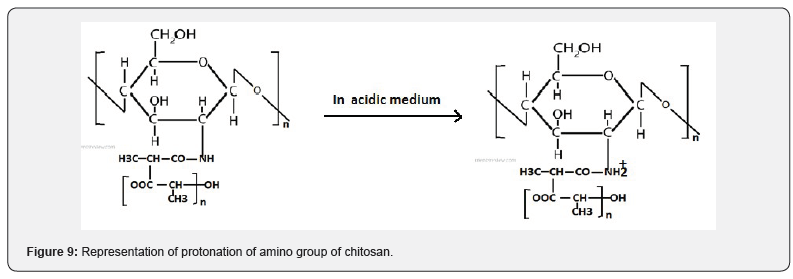
Effect of Temperature
The effect of variation of temperature on the swelling ratio has been examined at varying temperatures ranging between 15 to 45 0C. The observed results are presented in Figure 10, which reveal that the value of swelling ratio significantly rises at the particular level and then start over to decrease. It may be due to the large quantity of water which was penetrated into internal matrix of nanoparticles as a result of the enhanced rate of water diffusion. The resultant initial increase in the swelling of nanoparticles may be due to the large quantity of water which was penetrated into internal matrix of nanoparticles as a result of enhance rates of water diffusion and segmental mobility of macromolecular chains [37]. However, beyond 35 0C the swelling ratio was reduced, it may be due to the reason that at high temperature the hydrogen bonds get weaken between the solvent and polymer chains.
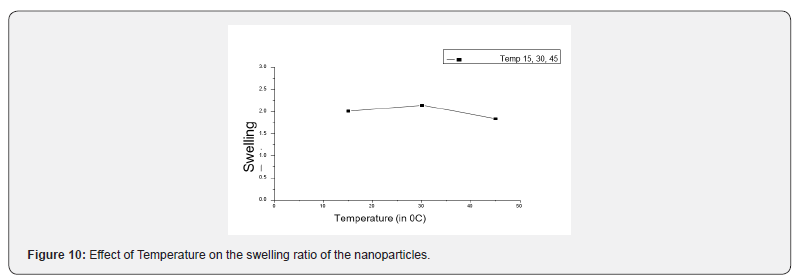
Effect of Physiological fluids
The swelling behaviour of nanoparticles in biological fluid was investigated by using urea, D-glucose, potassium iodide (KI), Phosphate Buffer Saline and the physiological fluids such as saline water. The observed results are presented in Figure 11, which reveal that the swelling behaviour was more in PBS than the other fluids. The possible reason for the swelling behaviour of nanoparticles in these solvents may be due to the presence of ions in the swelling medium which leading to an osmotic pressure differences between the biopolymer and the external solution. The swelling behaviour is influenced by the forces of interaction between solvent molecules and polymer [38].
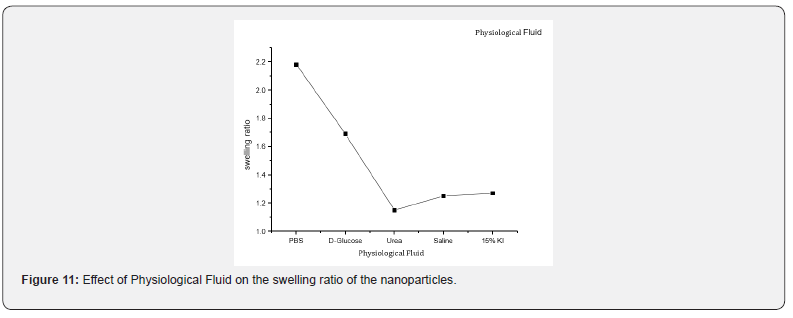
Effect of Chitosan Concentration
The swelling extent of nanoparticles on chitosan concentration is shown in Figure 12. When the quantity of chitosan is varied in the range of 0.1 g to 0.45 g in the synthesis of the nanoparticles, the swelling behaviour was significantly affected. The results are show that with an increase in the chitosan concentration their swelling behaviour increased and reaching the maximum value of swelling capacity. Which may be due to the fact that with increasing amount of chitosan, hydrophilicity of nanoparticles also increases. In addition, higher chitosan content enhances the hydrophilicity of the nanoparticles in chitosan-co-lactic acid causes a stronger affinity for more absorption of water. However, a further increase in monomer concentration (beyond 0.35 g), however results in decreased swelling. This is probably due to the reason that at much higher content of chitosan, the Nano spheres become morphologically and structurally dense that the decrease the diffusion of water molecules into the particles gets slowed down [39].
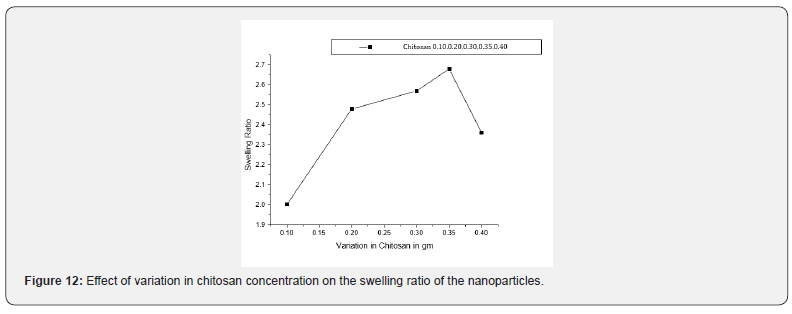
Effect of Variation in Lactic acid
The dependence of the swelling extent of nanoparticles on amount of lactic acid was investigated by varying the amount of lactic acid in the range of 1 ml to 4 ml in the feed mixture of the nanoparticles, the swelling ratio is significantly affected. The observed results are presented in Figure 13, which indicate that the swelling behaviour was decreases with increasing amount of lactic acid. The decreasing swelling ratio is due to the fact that with increasing amount of lactic acid hydrophobicity of nanoparticles also increases. In addition, higher lactic acid content enhances the hydrophobicity of the chitosan-co-lactic acid nanoparticles causes a weaker affinity for absorption of water. The amount of free water molecules is dependent on the nanoparticle structure that ultimately influences the swelling ratio. The results may be explained by the other fact that as the content of lactic acid increases in the nanoparticles, the polymeric nanoparticles becomes dense and compact, a compact nanoparticle structure contains lower quantity of free water [40].
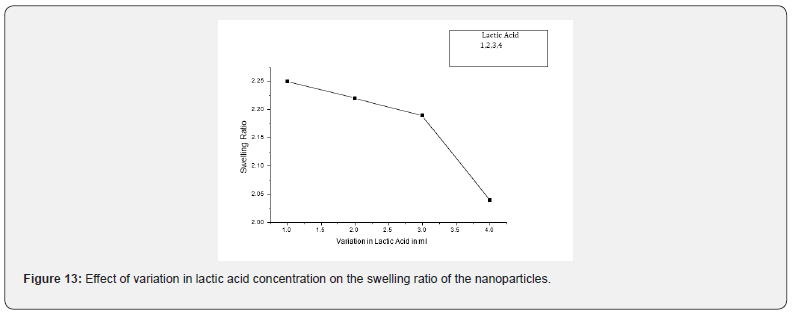
Loading of Drug
To determine the carrier capacity of nanoparticles with respect to drug, various batches of nanoparticles containing 0.50, 1.0, 1.5, 2.0, 2.5, 3.0 mg/ml of drug were prepared keeping the amount of nanoparticles as constant. It was observed that as the concentration of drug increases, loading of the drug also increases but after certain concentration the loading efficacy decreases due to saturation of the polymer matrix. The obtained results of cisplatin and 5-Fluoro uracil drug are shown in Tables 1 & 2.
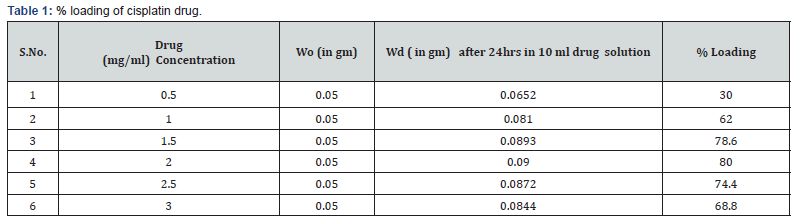
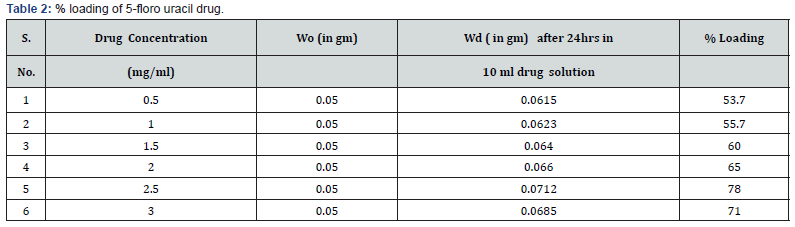
Encapsulation Efficiency
Various batches of nanoparticles containing different composition of biopolymer were prepared keeping the concentration of drug as constant. The initial concentration of drugs plays an important role in the encapsulation efficiency of chitosan-co-lactic acid nanoparticles. The obtained results are shown in Tables 3 & 4, which shows that the increase the concentration of chitosan in nanoparticles encapsulation efficiency also increases. It was found that the encapsulation and loading efficiencies of chitosan-co-lactic acid combination nanoparticle could be attributed to the fact that chitosan-co-lactic acid has a more electrostatic attraction with drugs [41]. % EE = (A – B/ A)* 100
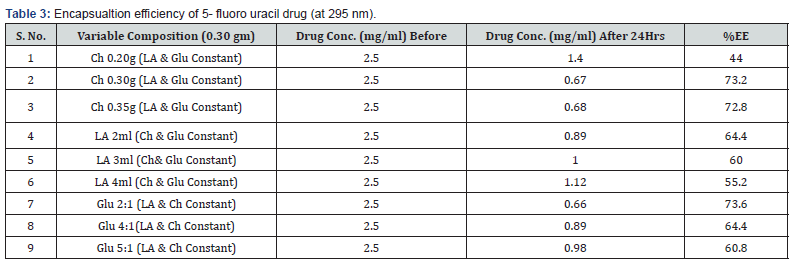
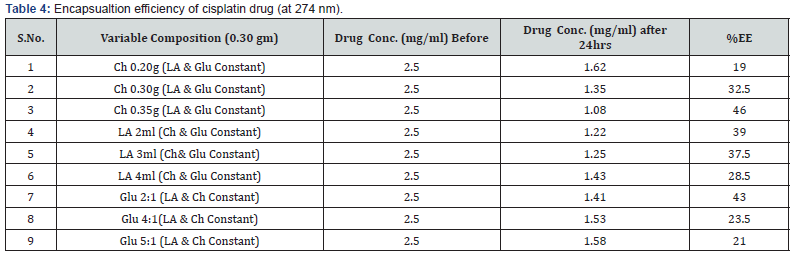
Invitro Cytotoxicity
The MTT Assay of L929 cells after contact with 50%, 25%, 12.5% and 6.25% extracts of Chitosan- lactic acid showed 9.51%, 9.87%, 27.79%, 43.28% metabolic activity respectively. Positive control showed 25.17% and negative control showed 93.17% metabolic activity.
Evaluation of Biocompatibility
The selection of a material to be employed as a biomaterial for a specific end use must meet several criteria such as favourable physicochemical properties, function desired, nature of the physiological environments, adverse effects in case of failure, expected durability and considerations relating to cost and ease of production. Whatever being the type of materials the biocompatibility is the foremost requirement for all biomaterials. In the present study, the assessment of in vitro biocompatibility has been made on the basis of two in vitro tests viz. BSA (bovine serum albumin) adsorption test, and heamolysis assay as discussed below:
BSA Adsorption
In the present investigation, the biocompatibility of prepared nanoparticles has been judged by monitoring the amount of protein (BSA) adsorbed by the nanoparticles. The results are shown in Table 5, which indicate that the amount of adsorbed BSA decreases with increasing amount of chitosan, in the feed mixture of nanoparticles. The observed results may be explained on the basis of fact that chitosan, is hydrophilic in nature and do not provoke either any damage to blood cells or the surface of plasma proteins which are the main requirement for biocompatibility. Furthermore, it is also known that protein adsorption is more favourable onto hydrophobic surface rather than hydrophilic one. Thus, an enhanced hydrophilic nature of nanoparticles with increasing content of chitosan results in lower protein adsorption.
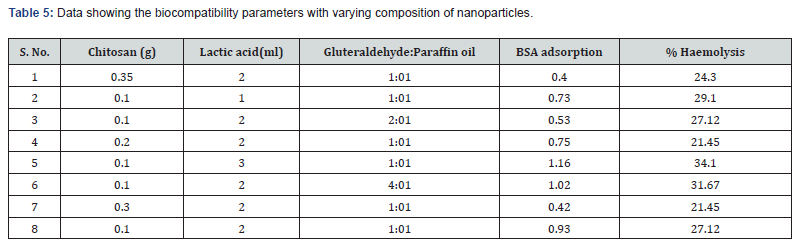
% Heamolysis Test
Heamolysis studies were performed on nanoparticles of various composition and the results are summarized in Table V, which indicate that with increasing chitosan content, the extent of heamolysis decreases. The observed results may be attributed to the reason that with change in chitosan concentration in the feed mixture of the nanoparticles, the surface composition favourably changes, which improves the blood compatible quality of the material.
Conclusion
Nanoparticles of chitosan and lactic acid were prepared by emulsion crosslinking method and characterized by various analytical techniques like FTIR spectroscopy, SEM and TEM, which confirmed the functional groups of nanoparticles, surface and size of nanoparticles. The swelling behavior of Nanoparticles was investigated in this study. It was noticed that the water sorption property was dependent on the chemical composition of nanoparticles of chitosan-co-lactic acid. With the increase in amount of chitosan up to 0.35 g, the adsorption of PBS increased, whereas, a further increase in chitosan above 0.35 g, resulted in a fall in swelling ratio. On increasing the amount of lactic acid content, the degree of swelling ratio was decreased. The extent of water sorption by these nanoparticles was found to increase from neutral to acidic pH and decrease in alkaline pH at room temperature (30 0C). A lower value of degree of swelling was observed in simulated biological fluids like saline water, KI solution and D-glucose solution. The nanoparticles were characterized by various analytical techniques like FTIR, SEM and TEM, which clearly showed the presence of various functional groups present on the nanoparticles of chitosan-co-lactic acid, morphology and the size of nanoparticles. It was observed that the water imbibition capacity was dependent on the chemical composition of the nanoparticles. The current approach is mainly based on the entrapment of an anticancer drugs into the nanoparticles to be protected and transported by the bloodstream. Polymeric nanoparticles do not provide any benefit in the treatment of disease, but rather they act as carrier for the drugs. In the present studies the addition of model drug, cisplatin and 5-fluoro uracil to polymeric nanoparticles results in 46% and 73.6% drug entrapment respectively. Also, the assessment of biocompatibility has been made on the basis of two in-vitro test viz BSA adsorption test and haemolysis assay. These assessment is indicating that these nanoparticles are suitable for biomedical application.
For more Open Access Journals in Juniper Publishers please click on: https://juniperpublishers.com/
For more articles in Academic Journal of Polymer Science please click on: https://juniperpublishers.com/ajop/index.php
Comments
Post a Comment