Deep Eutectic Solvents in The Synthesis of Polymers-Juniper Publishers
JUNIPER PUBLISHERS- ACADEMIC JOURNAL OF POLYMER SCIENCE
Abstract
Deep Eutectic Solvents (DESs), a subclass of ionic
liquids, have emerged as sustainable and green solvents in chemical
reactions. In this mini-review article, deep eutectic solvents
utilization in the polymer synthesis will be discussed briefly.
Keywords: Deep eutectic solvents; Polymer synthesis; Green chemistry
Abbrevations:
DES: Deep Eutectic Solvents; HBA: Hydrogen Bond Acceptor; HBD: Hydrogen
Bond Donor; PDCs: Poly Diol-Co-Citrates; PAAc: Poly (Acrylic Acid);
PMAAc: Poly Methacrylic Acid; APS: Ammonium Persulfate; EM: Eutectic
Mixture; FP: Frontal polymerization
Introduction

Solvent utilization creates the largest volume of
auxiliary waste in polymer synthesis. However, increasing awareness of
the environmental problems has led to the use of alternative reaction
media to reduce or eliminate organic solvent use. Deep eutectic solvents
(DESs) are systems formed from a eutectic mixture of Lewis or Bronsted
acids and bases which can contain a variety of anionic and/or cationic
species [1]. They incorporate a hydrogen bond acceptor (HBA) and a
hydrogen bond donor (HBD), which are able to give a eutectic with a
melting point much lower than either of the individual components
(Figure 1) [2]. One of the most significant deep eutectic phenomenon’s
was observed for a mixture of choline chloride and urea in a 1:2 mole
ratio respectively. The resulting mixture has a melting point of 12°C,
which makes it liquid at room temperature. DESs are obtained by
complexion of quaternary ammonium salts with hydrogen bond donors. The
charge delocalization occurring through hydrogen bonding between the
halide anion and the hydrogen donor
moiety is responsible for the decrease in the freezing point of the
mixture relative to the melting points of the individual components
(Figure 2) [3].
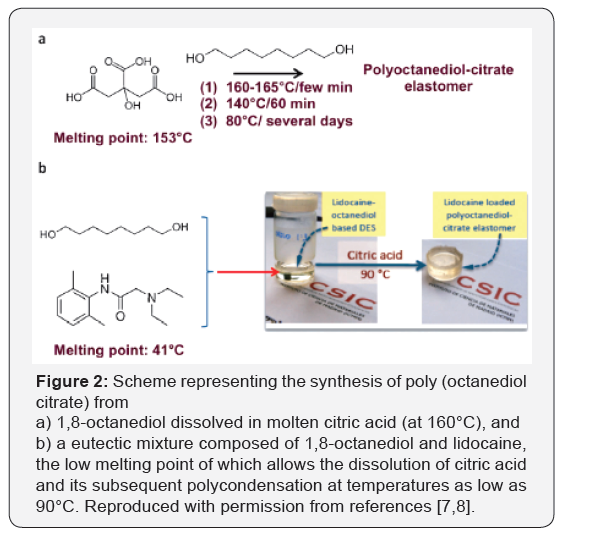
DESs share many characteristics of conventional ILs
(e.g. non-volatile, nonreactive with water, and biodegradable) while it
offers certain advantages. The preparation of eutectic mixtures in a
pure state can be accomplished more easily than that of ILs with no need
of post-synthesis purification due to the purity of the resulting DES
will simply depend on the purity of its individual components. Moreover,
the low cost of those eutectic mixtures based on readily available
components (for instance, urea and choline chloride are a well-known
example) makes DESs share many characteristics of conventional ILs (e.g.
non-volatile, nonreactive with water, and biodegradable) while it
offers certain advantages. The preparation of eutectic mixtures in a
pure state can be accomplished more easily than that of ILs with no need
of post-synthesis purification due to the purity of the resulting DES
will simply depend on the purity of its individual components. Moreover,
the low cost of those eutectic mixtures based on readily available
components (for instance, urea and choline chloride are a well-known
example) makes
Green Polycondensations
The polycondensation of citric acid with 1,8-octanediol for the
synthesis of Poly (Diol-Co-Citrates) (PDCs), were first reported
by Yang et al. [5,6] in 2004 and, similar to other biocompatible
polyesters, have indicated tremendous versatility as polymeric
networks for regenerative medicine. The conventional synthetic
process first consists of citric acid melting by thermal treatment
at 160-165°C for a few minutes (Figure 1). Then, 1,8-octanediol
is dissolved in this molten phase and polycondensation starts.
The temperature is maintained at 140°C over 60 min, and then
reduced to 80°C to let the reaction to proceed over several days
until completion. Deep eutectic solvent-assisted syntheses offer
an interesting opportunity to fits well with green chemistry.
For example, the mixture of 1,8-octanediol and lidocaine, which
is a local anesthetic, in stoichiometric molar ratios forms a
eutectic with a melting point of about 40°C. Citric acid can
easily be dissolved in this eutectic mixture and polycondense
with 1,8-octanediol at temperatures far below those described
above for regular polycondensations (Figure 2). Lidocaine was
finally entrapped with a high loading due to the stoichiometric
in which it formed part of the eutectic mixture into the resulting
polyesters. Thus, we were able to obtain drug-eluting materials,
the controlled release of which into an aqueous medium was
simply based on the biodegradable character of the polyester
network. It is worth noting that lidocaine would decompose (at
least, partially at 155°C) following regular polycondensation
[7,8].
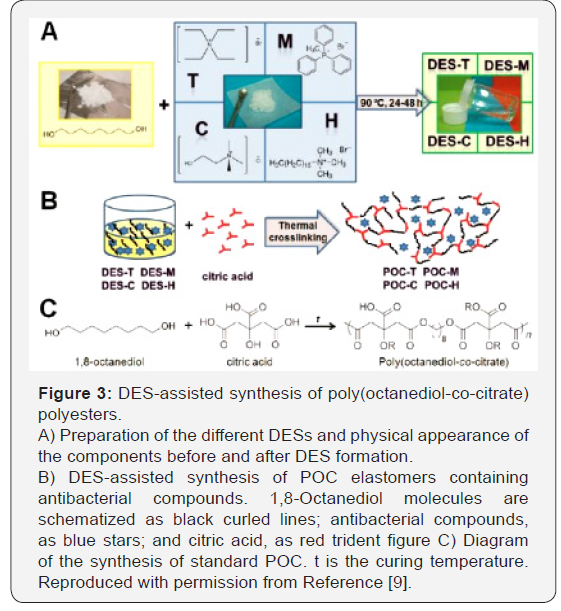
Also, Serrano et al. [9] in another research, prepared
biodegradable poly(octanediol-co-citrate) polyesters with
acquired antibacterial properties by the DES-assisted
incorporation of quaternary ammonium and phosphonium salts
into the polymer network (Figure 3) [9]. Remarkably, the resulting
polymers preserve their cytocompatibility while showing elastic
properties advantageous for their use as wound dressings. In the
resulting polymers, the presence of salts such as: choline chloride,
tetraethylammonium bromide, hexadecyltrimethylammonium
bromide, and methyltriphenylphosphonium bromide, inhibits
bacterial growth in the early post implantation steps, as tested
in cultures of Escherichia coli on solid agar plates.
From DSC scans, the melting points (Tm) for DES-C, DES-T,
DES-H, and DES-M were identified at 52, 47, 54, and 55°C,
respectively, which were significantly below those of any of its
individual components (i.e., 61°C for 1,8-octanediol, 302-305°C
for compound C, 285°C for T, 248−251°C for H, and 230−234°C
for M) (Figure 4). Hydrogen bonding between the halide anion of
quaternary nitrogen or phosphonium salts and the 1,8-octanediol
as the hydrogen donor moiety is the most plausible explanation
for the stabilization of the different DESs formed, as previously
described for other DESs [4,7].

Polymer inhibition of bacterial growth against E. coli was
measured as the diameter of the zone of inhibition around
polymer discs normalized by the grams of polymer. A clear zone
of inhibition was observed around the discs, with values varying
from 1.76 (POC-C) to 68.90 (POC-M) mm g-1 of polymer as the
minimum and maximum inhibition zones, respectively.
DESs have been used in polycondensations, where one
of the components was also a reactant, thus called all-in-one
systems [10]. Electrochemical polymerization of conductive
monomers has also been explored taking advantage of the ionic
conductivity of DESs [11-15], including the FeCl3-catalyzed
oxidative polymerization of 3-octylthiophene [14]. Recently,
DESs were reported to play the role of curing agents in epoxy
resins [16], monomer [17,18] and initiator [19] in ring-opening
polymerization.
Free-radical polymerization of DESs: deep eutectic monomers
In free-radical polymerization of DESs, monomers able to
polymerize undergo free-radical polymerization while taking
part in a DES, as hydrogen bond donor or ammonium salt, so
called DES monomers [20]. The introduction of DESs in freeradical
polymerizations was reported by the synthesis of Poly
(Acrylic Acid) (PAAc) and Poly (Methacrylic Acid) (PMAAc)
monoliths by frontal polymerization [21]. Frontal polymerization
(FP) is normally performed with neat monomers but can be
accomplished in solution [22-25]. Bednarz et al. [26], described
the free radical copolymerization of itaconic acid−forming DES
with ChCl−and AAm by in situ polymerization-crosslinking of the
DES (Figure 5). Itaconic acid is a bio-based monomer containing
an unsaturated moiety that slowly polymerizes by free radicals;
it also has two carboxylic groups available as HBDs [27]. As result
of Itaconic Acid (IA) transformation into DES, copolymerization
initiated by persulfate proceeded faster and at lower
temperature than in water and produced higher crosslinked
hydrogels, pointing to a catalytic effect of ChCl. Based on the
results of itaconic acid free-radical polymerization initiated by
Ammonium Persulfate (APS) in water, it was concluded that
DES acted as the solvent of itaconic acid and also as catalyst in
free-radical polymerization processes [26]. According to this
study, the copolymerization-crosslinking of itaconic acid with
N, N’-methylenebisacrylamide in the DES was a useful method
for the preparation of hydrogels. Comparative studies indicate
that itaconic acid undergoes faster copolymerization in DES than
in water, and probably for that reason the obtained product has
higher cross-link density [26].
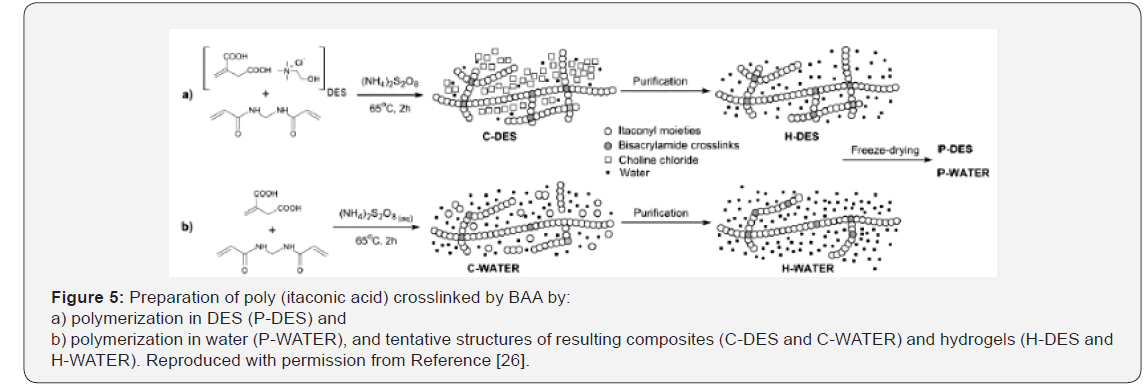
DES assisted atom transfers radical polymerization

A green solvent system, composed of a eutectic mixture
(EM) and ethanol, was used in the supplemental activator and
reducing agent atom transfer radical polymerization (SARA
ATRP) of methyl acrylate (MA), yielding well‐defined polymethyl
acrylate (PMA) chains (Figure 6) [27]. In addition, this eutecticbased
system allows a straightforward separation of the entire
catalytic system, with possibility of reutilization, which is a much
advantageous feature for a possible industrial implementation
of the SARA ATRP process [27]. In this study, ethanol/reline
(choline chloride: urea) mixtures showed to be excellent solvent
for the polymerization, providing well-defined PMA (dispersity,
Ð(Mw/Mn) <1.2) as well as affording the recycling of the entire
catalytic system Cu(0)/CuBr2 /Me6TREN, which could be reused
at least two times in new SARA ATRP experiments.
Conclusion
Deep eutectic solvents provided a reaction medium, so
that polymerizations were ultimately performed in a solventless
manner. DESs also provided new precursors that favored
more efficient polymerizations by decreasing the energy input
required for the reaction to proceed. The use of mild reaction
conditions in combination with the compositional versatility of
DESs, which allows the selection of low toxic components, is also
of interest from the viewpoint of green chemistry due to it opens
up the way to the design of eco-friendly synthetic methods. DESassisted
polymer syntheses met more green principles than
those of conventional ones, so, polymers obtained from greener
processes have more opportunities to be feasible for green
applications than polymers obtained from less green ones. These
encouraging results suggest that DES-assisted polymerizations
can help to provide synthetic designs and products with a
greener character.
For more articles in Academic Journal of Polymer Science please click on:https://juniperpublishers.com/ajop/index.php
Comments
Post a Comment