Polymer Enhanced Oil Recovery Process: An Updated, Narrowed Review-Juniper Publishers
JUNIPER
PUBLISHERS- Academic Journal of Polymer Science
Abstract
Application of polymers and nanoparticles have been
suggested by several researchers as a chemical enhanced oil recovery
(EOR) agent in carbonate reservoirs each of which has a specific
influence on the reservoir rock and fluid properties. However, the high
salinity of the reservoir condition along with high temperature has a
detrimental effect on the performance of both polymers and
nanoparticles. Common polymers and nanoparticles are usually degraded
and begin to aggregate with the salinity increment. The purpose of this
research is to provide a comprehensive review on the combination of
general/thermoassociative polymers with low salinity water and
nanoparticles as three new approaches for the chemical EOR process. The
most previous studies have been concerned application of polymers and
nanoparticles in the EOR, recommending usage of thermoassociative
polymers (resistant under high salinity and high temperature conditions)
as a substitution of common viscosifiers, and also as a good stabilizer
of nanofluids to provide a higher stability for nanoparticles. All
makes new polymer materials as a promising candidate for offering some
ideas to see the polymer complexity world and its pros/cons as a one of
the promising EOR agent.
Keywords: General polymer; Tanoparticle; Thermoassociative polymers; EOR processAbbrevations: EOR: Enhanced Oil Recovery; IFT: Interfacial Tension; PAM: Polyacrylamide; HPAM: Hydrolyzed PAM; HSHT: High Salinity and High Temperature; LoSal: Low Salinity; CTAB: Cetrimonium Bromide; SDS: Sodium Dodecyl Sulfate; CMC: Critical Micelle Concentration; PVP: Polyvinylpyrrolidone; PPNs: Polyacrylamide Nanoparticles; PSt: Polystyrene; TAP: Thermo Associative Copolymers
Introduction
Carbonate reservoirs account for more than 60% of the
world’s oil. However, due to the presence of the fracture network and
oil-wet matrix, oil recovery from naturally fractured carbonate
reservoirs has always been a challenging issue. Hence, application of
EOR methods especially chemical EOR has been recently gained a lot of
attention by researchers to obtain a higher recovery of carbonate
reservoirs. Application of polymers and nanoparticles are a good example
of chemical agents each of which has a specific effect on the reservoir
rock and fluid properties. The govern mechanism of polymer flooding is
the viscosity enhancement of aqueous solution, while nanoparticles lead
to the Interfacial Tension (IFT) reduction and wettability alteration of
reservoir rocks. Common polymers such as Polyacrylamide (PAM) and
Hydrolyzed PAM (HPAM) usually exhibit a high degree of chemical
degradation against High Salinity and High Temperature (HSHT) reservoirs
which results in the solution viscosity reduction with the salinity and
temperature increment [1]. Hence, various researches have been
conducted concerning application of Low Salinity (LoSal) water with
polymers in order to mitigate the detrimental effect of high
salinity [2-4]. High salinity also has a worse effect on nanofluids
stability [5]. However, application of thermoassociative polymers which
are stable in high salinity and high temperature conditions, can be a
good agent compensate for the effect of high salinity on polymers and it
can also be applied as a stabilizer for nanofluid solutions to obtain a
higher stable nano-suspension.
In this paper, a comprehensive review of the most
recent studies has been conducted in order to analyze the application of
the polymers combination with low salinity water, nanoparticles, and
combination of nanoparticles (NPs) and thermoassociative polymers in EOR
process.
Results and Discussion
Combination of Polymers with Low Salinity Water
Shiran et al., [2], investigated the effect of the
low salinity water and hydrolyzed HPAM on the oil recovery and observed
an increment in the total recovery which is mainly due to the
combination effect. Vermolen et al., [1], expressed these combination
effects and claimed that combination of the LoSal/polymer will improve
the oil sweep efficiency through the trapped oil mobilization which are
detached from the rock surface due to the wettability alteration. In
order to precisely
shown the effect of brine salinity on polymer solution viscosity, a
semi-log diagram of polymer viscosity vs. brine salinity is shown
in Figure 1. As it is obvious, two plateau ranges are observed
where the reservoir brine salinity is in the ranges of lower
plateau, while low salinity brine is on the steep part of the graph
in which the polymer viscosity is highly sensitive to the brine
salinity. They concluded that the amount of the required polymer
for obtaining a target viscosity is lowered by the usage of LoSal
which is favorable for economic considerations. Furthermore,
they observed an increase in the stability of polymer at the
high temperatures when it is combined with LoSal confirming
the application of LoSal/polymer at high salinity and high
temperature conditions.
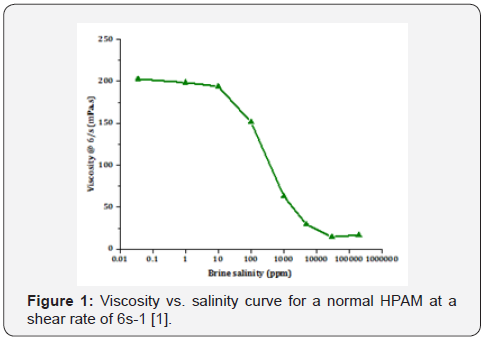
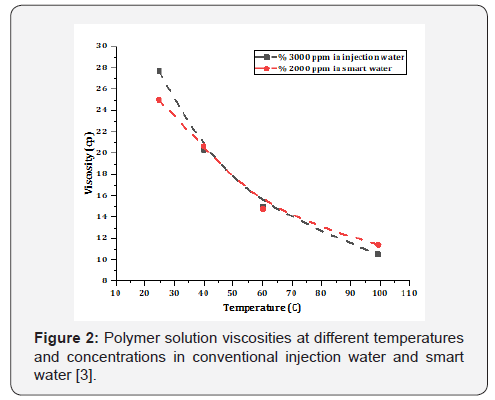
Recently, AlSofi et al., [3] have investigated the simultaneous
usage of a sulfonated polyacrylamide polymer with the smart
water (salinity of 10 times lower than the injected water) and
they observed the required polymer concentration for achieving
a specific viscosity is reduced (from 3,000ppm to 2,000ppm)
in the case of smart water instead of high salinity ones (Figure
2). Besides, core-flooding experiments have shown a significant
increment in the oil recovery by smart water/polymers
comparing to the separate cases. The effect of LoSal combination
with the polymer on controlling polymer gel blocking in the
fractured reservoirs have been analyzed by Brattekas et al. [4].
They observed that the gel fracture blocking is improved via
LoSal as a chase water. Hence, the fractures will be successfully
blocked to divert the flow direction toward matrix blocks.
Standalone Nanoparticles for EOR Purposes
Due to the unique properties of nanoparticles, their
applications have been recently suggested as a promising EOR
method. NPs have small size (1 to 100nm) assisting them to flow
through a typical reservoir pore spaces (lower than 1micron).
Besides, their surface properties can be manipulated in order to
become more appropriate for desirable application [6]. In order
to get insight into the actual mechanisms by which NPs improve
the oil recovery from reservoirs, a number of researches have
been conducted that some of them are explained below.
SiO2: The applicability of hydrophilic SiO2 NPs on the
efficiency of Cetrimonium Bromide (CTAB) as a cationic
surfactant has been analyzed by Ravera et al. [7]. A reduction
in the IFT was observed due to the adsorption of positively
charged CTAB on the surface of silica NPs (in 1wt% nanosuspension).
In another research conducted by Ma et al. in 2008,
the efficiency of Hydrophilic SiO2 NPs was also analyzed on the
performance of Sodium Dodecyl Sulfate (SDS) as an anionic
surfactant and Triton X-100 as a non-ionic surfactant. They
observed hydrophilic SiO2 NPs improve the influence of SDS in
the IFT reduction which may be due to the presence of repulsive
forces between negatively charged SDS and SiO2 NPs. However,
they do not affect the performance of Triton X-100 because of
existing poor interactions [8]. Zargartalebi et al., [9] investigated
the influence of hydrophilic and slightly hydrophobic SiO2 NPs
(0.2wt%) on the SDS performance, observed a reduction in the
surfactant adsorption on the sandstone surface in the presence
of NPs especially for hydrophobic ones. Besides, they claimed
that the influence of NPs on the IFT reduction was completely
dependent on the surfactant concentration. As it is illustrated
in Figure 3a and Figure 3b, at low surfactant concentration up
to Critical Micelle Concentration (CMC), IFT values continuously
reduced. However, above CMC value, the IFT began to increase
and finally reached to a constant value even lower than the one
obtained by surfactant alone.
The ability of silane treated SiO2 and hydrophobic SiO2 NPs
(with a concentration of 0.3wt% dispersed in ethanol) in the
wettability alteration of reservoir rock has been firstly observed
by Ogolo et al. in 2012 [10]. After a year, Li et al. confirmed the
high efficiency of hydrophilic SiO2 NPs in both IFT reduction
and wettability alteration of sandstones in the presence of
30,000ppm NaCl solution with 0.05wt% NPs, showing an
optimum concentration [11]. Furthermore, Roustaei et al., [12]
investigated the potential of hydrophilic SiO2 NPs in the presence
of 50,000ppm NaCl solution in EOR of carbonate rocks and
they concluded that at an optimum concentration of 0.4wt%,
maximum wettability alteration is obtained. They also claimed
that NPs result in increasing IFT when the wettability is altered toward water-wetness and this IFT increment contributes in
the oil recovery improvement. Their assertion is based on the
concept of spontaneous imbibition in carbonate reservoirs.
Recently, the effect of temperature and size of SiO2 NPs has been
analyzed by Al-Anssari et al., [13] and they concluded that the
temperature has a positive effect on SiO2 NPs ability to alter
wettability of oil-wet carbonate rocks; however, the effect of NPs
size is negligible.
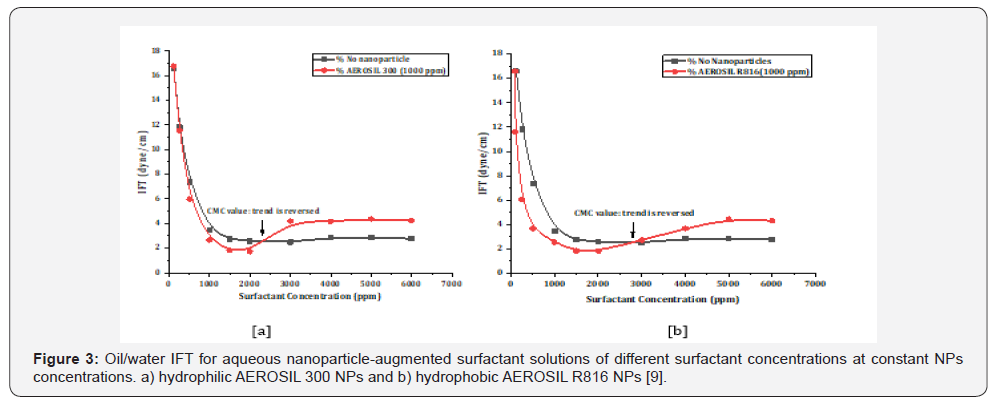
ZrO2: Karimi et al., [14] investigated the process of
spontaneous imbibition of 5wt% ZrO2 nanofluid into an oil-wet
carbonate reservoir. They claimed that adsorption of hydrophilic
synthesized ZrO2 NPs and the mixture of nonionic surfactants
onto the rock surface leads to the formation of a nano-textured
surface which can modify reservoir rock wettability toward
more water-wetness better than NPs alone and consequently
more oil is recovered.
Al2O3: Ogolo et al., [10] claimed that Al2O3 NPs (dispersing in
distilled water or brine with a salinity of 30,000 ppm) perform
well in EOR due to the oil viscosity reduction. However, a year
later, Giraldo et al., [15] observed that Al2O3 can also alter the
wettability of sandstones from severe oil-wet to severe waterwet
when synthesized by an anionic surfactant. Among a wide
range of nanofluid concentration between 0.01 to 1wt%, the
efficiency of surfactant to wettability modification is improved
just at a relatively low NPs concentration (equal or lower than
0.05wt%). Besides, they asserted that imbibition experiments
are a good evaluation tool for analyzing the performance of NPs.
The ability of Al2O3 NPs for IFT reduction is expressed by Joonaki
et al., [16] and they observed that the interfacial tension between
phases began to reduce when propanol is used as a dispersing
agent for Al2O3 NPs.
TiO2: Ehtesabi et al., [17] investigated the application of
synthesized TiO2 NPs through core-flooding experiments in
sandstone rocks and they confirmed their application in EOR
in terms of wettability alteration and oil viscosity reduction.
They observe that TiO2 NPs do not affect the oil viscosity but
in a low concentration of 0.01wt% could alter significantly
the wettability of rock surface. Application of SiO2, Al2O3 and
TiO2 NPs in oil-wet carbonate reservoirs have been analyzed
by Esfandyari et al., [5] under various temperatures (26, 40,
50, 60 ℃). The maximum and minimum reduction in contact
angles were achieved by SiO2 and Al2O3 NPs, respectively at all
temperatures. Besides, they observed a reduction in oil viscosity
at higher temperatures of 50 and 60 ℃ by Al2O3 and TiO2 NPs
and the oil recovery by Al2O3. TiO2 was also more significant at all
temperatures comparing to SiO2 NPs. The potential of these three
hydrophilic NPs has been also investigated by Hendraningrat et
al. in different wettability sandstone reservoirs. They confirmed
the mechanism of wettability alteration as the main mechanism
of these NPs although IFT reduction is also observed. As a result
of their experiments, TiO2 NPs performed well in all types
of wettability systems among other NPs [18]. Generally, the
mechanism by which NPs alter the reservoir rock wettability is
due to the creation of a wedge-film on the rock surface due to the
disjoining pressure enhancement between oil and rock surface
(Figure 4). Consequently, the oil droplets will detach from the
rock surface and a new underlying layer is exposed with a more
water-wetness wettability [19].
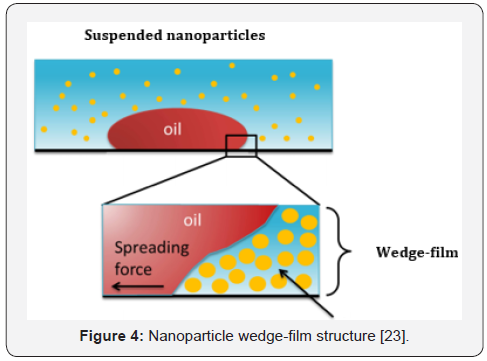
Stability of Nanoparticles
One of the main challenges of nanofluids which limits their
application especially in long period is their stability. Forming
an agglomeration may restrict the flow of NPs through micronsized
pore spaces and may lead to pore spaces plugging and
permeability reduction [18]. Zeta potential of nanofluid has
a direct relationship with the suspension stability in a way
that the suspension with higher zeta potential (even positive
or negative) shows higher stability. The water chemistry such
as pH, ionic strength (salinity) and its various component
usually influence the stability of nanofluids [20]. Accordingly,
it is expected that adjusting the pH value of system, reducing
its salinity or adding a stabilizer to the nano-suspension will
provide a higher stability for system. Adjusting pH value: The pH
value of a nanofluid solution influence their surface charge and
consequently their stability. According to Huang et al. there exist
an optimal pH value by which a maximum nanofluid stability is
obtained. They have conducted a series of experiments on Al2O3
and CuO nanofluids in which the pH value of the solution is
adjusted by addition of HCl or NaOH to obtain an acidic or basic
suspension. They observed that Al2O3 and CuO nano-suspension
obtain the highest stability at an optimum pH value of 7.5- 8.9
and 7.5-9.5, respectively [21].
Effect of salinity: Esfandyari et al., [5] measured the zeta
potential of the nanofluid of Al2O3, TiO2 and SiO2 in various
dispersion media of deionized water, NaCl solution (3,000
ppm) and synthetic brine (25,000ppm). As a result of their
experiments, the zeta potential values of SiO2, Al2O3 and TiO2
in deionized water are -38.5, 31.1 and -19.1mV, respectively
indicating the highest to lowest stable nanofluids. However,
the zeta potential values of SiO2, Al2O3 and TiO2 nanofluids have
been decreased to -32.3, 27.4 and -15.8 in NaCl solution and
to -22.4, 21.6 and -9.9 in the synthetic brine respectively, all of
which indicate the detrimental effect of salinity on the stability
of nanofluids.
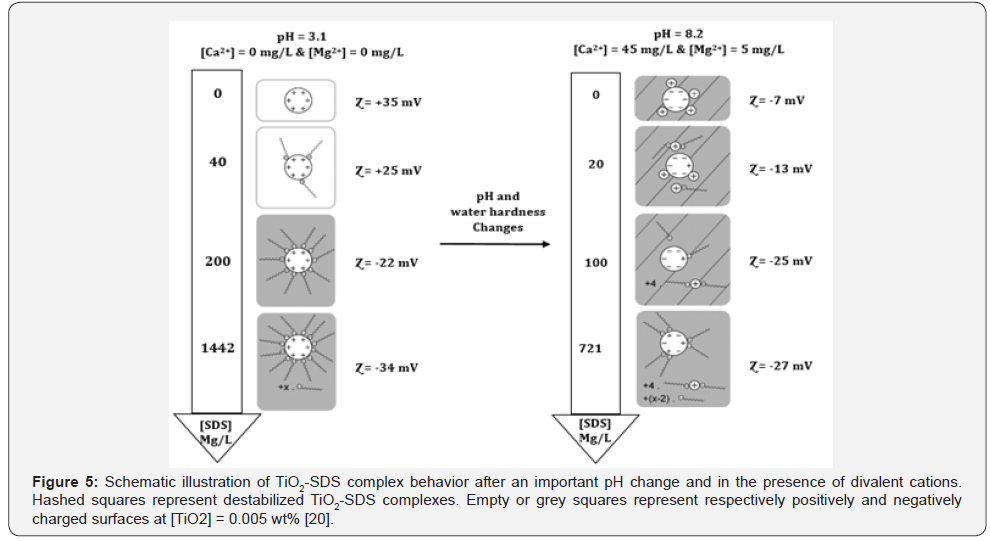
Adding stabilizer: Hendraningrat et al., [18] analyzed the
stability of nanofluid of Al2O3, TiO2 and SiO2 NPs. Analysis of
nanofluids visual stability show that Al2O3 NPs precipitated
at initial stages (approximately after 3 hs) and TiO2 NPs
precipitated slightly later. However, SiO2 NPs exhibit a better
stability of 24-48hrs. In order to get longer stability of Al2O3 and
TiO2 NPs, they proposed addition of Polyvinylpyrrolidone (PVP)
as a stabilizer to alter NPs surface conductivities, resulting in 96
and 48h stability for Al2O3 and TiO2 nanofluids, respectively. The
influence of pH value, existence of divalent cations presented
in water and surfactant concentration as a stabilizer on TiO2
nanofluid stability have been analyzed by Loosli et al. [20].
They observed that when the pH values are altered from pH=3
(addition of HCl) to pH=11 (addition of NaOH) the zeta potential
continuously decreased from +36mV to -40mV. At a pH range of
5-7.2, the absolute zeta potential values are minimum indicating
the range of instability. Zeta potential is equal to zero at a pH
value of 6.1, indicating the most instable situation. Besides,
they also concluded that the presence of divalent cations (Ca2+
and Mg2+) leads to the more agglomeration of NPs due to the
cation bridging. In contrast, they claimed that adsorption
of negatively charged sodium dodecyl sulfate, as an anionic
surfactant, onto the positively charged surface of TiO2 NPs make
the nano-suspension more stable. The influence of pH changes
and the presence of divalent cations on the stability of TiO2-SDS
complexes are illustrated in Figure 5.
Thermoassociative polymers
In order to prevent chemical degradation of commercial
PAM during EOR in high salinity and high temperature
conditions, Tamsilian et al., [22] have synthesized a protected
Polyacrylamide Nanoparticles (PPNs) in which a hydrophobic
polystyrene (PSt) sell is created by one-pot two-step inverse
emulsion surface polymerization method. The existence of
hydrophobic PSt shell protects the active PAM chains from
degradation in harsh reservoir conditions. Besides, the efficiency
of PPNs as a viscosity modifier is increased by their timedependent
releasement; hence, it will remain active in a wide
range of approximately 30 days. A schematic of PPNs polymer
flooding is illustrated in Figure 6. PPNs have a high potential
of IFT reduction and wettability alteration in comparison with
common PAM and according to the results of incremental oil
recovery, an average of 6.9% and 5.95% was obtained by PPNs
and PMA, respectively; which indicates PPNs better capability
in oil recovery improvement. Finally, a comparison between
the performance of PAM and PPN introduces PPNs as a good
candidate for EOR processes, due to their high capability in
viscosity increment even harsh conditions and also IFT
reduction and wettability alteration although a lower amount of
it is required for achieving same recovery.
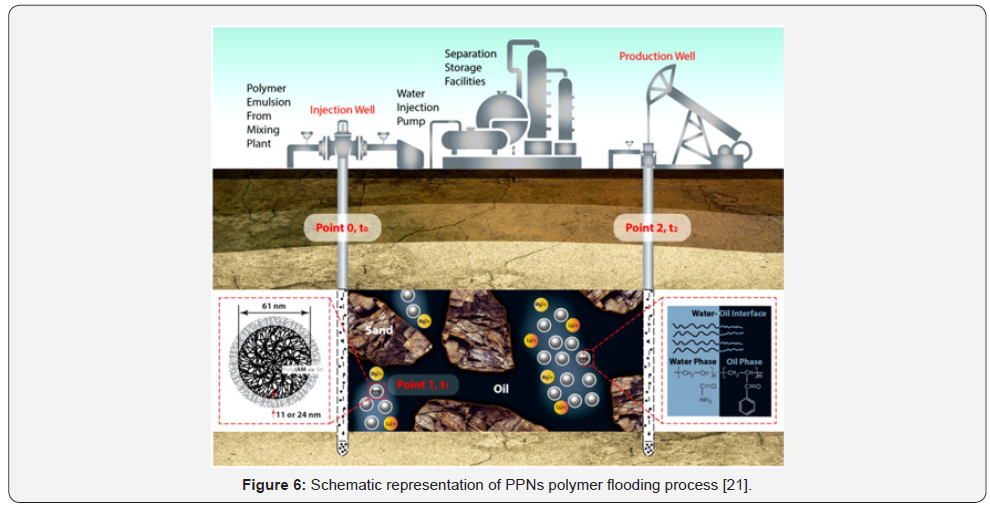
Shaban et al., [23] have synthesized a new cauliflowerlike
amphiphilic copolymer by aerosol-photopolymerization
method. A schematic of their experimental setup consists of an
atomizer, a flow-through photoreactor, and a collection filter
is shown in Figure 7. The advantage of this method over usual
methods such as emulsion polymerization is that no surfactants
are required. They concluded that their new method of synthesis
has a great ability to produce novel copolymers with large
amount of hydrophobic and hydrophilic monomers. Hence, it has
a long applicability to viscosity enhancement during the process
of polymer-flooding.
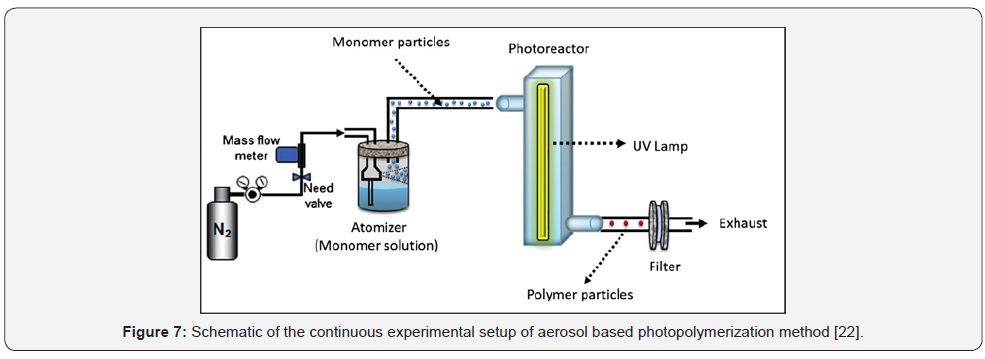
Another new type of acrylamide-based Thermo Associative
Copolymers (TAP), applicable in high salinity and high
temperature reservoirs, have been considered by the current
research community, called Nano Chemical Group, through the
process of a copolymerization mechanism of free-radical of
acrylamide monomer and thermosensitive macromonomers.
In a saline reservoir, the viscosity of a solution is augmented
if the temperature is above a critical value called associating
temperature. Meanwhile, it is expected that addition of a type
of stabilizer which is highly resistant in high salinity conditions
could provide a desired stability for nanofluids at high salinity
conditions. Considering this in mind, the novel hydrophobically
thermoassociating copolymer (HTAP) consisting of
polyacrylamide and polystyrene, prepared by Tamsilian et al.,
[22], which has been recently used as EOR agent is recommended
to stabilize NPs to compensate for the detrimental effect of high
salinity.
Conclusion
In this study, a comprehensive review has been conducted
on the combination of polymers with low salinity water,
nanoparticles, and thermo associative polymers, providing three
new approaches for the polymer EOR process. The following
conclusions can be obtained from the reviewed researches:
I. Due to the negative impact of the salinity on the
efficiency of polymer, application of low salinity water with
common polymers mitigates its worse effect, resulting in more
oil recovery. Besides, the required polymer amount for achieving
the same viscosity is lower comparing to the cases in which high
salinity water is used.
II. SiO2 nanoparticles results in more oil recovery by the
wettability alteration of the reservoir rock and IFT reduction.
ZrO2 and TiO2 nanoparticles by wettability alteration and Al2O3
nanoparticles by IFT reduction, wettability alteration and oil
viscosity reduction lead to oil recovery improvement all of which
are among the effective nanoparticles.
III. The stability of nanofluids is affected by the water
chemistry such as pH, ionic strength (salinity) and its various
components. There exists an optimum value of pH by which
maximum stability is obtained for each nanofluid. Salinity has
usually a detrimental effect on nanofluids stability and result in
their agglomeration.
IV. Thermoassociative polymers which their efficiency
remain stable at the high salinity and high temperature
conditions, are suggested as a promising substitution for
common polymers and also as a stabilizer of nanofluids at high
salinity harsh conditions.
V. Reviewing these three aspects of polymer applications
in the EOR process shows a good thought streamline for research
groups who are active to see the polymer complexity world and
its pros/cons as a one of the promising EOR agent.
For more articles in Academic Journal of Polymer
Science please click on:
https://juniperpublishers.com/ajop/index.php
https://juniperpublishers.com/ajop/index.php
Comments
Post a Comment