Nickel and Palladium Catalyzed Olefin Polymerization-Juniper Publishers
JUNIPER
PUBLISHERS- ACADEMIC JOURNAL OF POLYMER SCIENCE
Abstract
This review summarized the progress on transition
metal catalysts for olefin polymerization, and focuses on the olefin
polymerization by the late transition metal catalysts and the recent
advances in catalyst design. In recent years, nickel and palladium
complexes for olefin polymerization have received extensive researches
owing to the precise control of branching microstructure, molecular
weights and properties of the resulting polyolefins.
Keywords: Nickel and palladium complexes; Olefin polymerization; Chain-walking; Branched polymer
Abbrevations:
MAO: Methylaluminoxane; CGC: Constrained Geometry Catalysts; LDPE: Low
Density Polyethylene; LLDP: Linear Low-Density Polyethylene; HDPE:
High-Density Polyethylene; PP: Polypropylene
Introduction
The research of the ethylene and α-olefins
polymerizations using late transition metal catalysts should be
highlight for development of polyolefin materials during the past two
decades [1-5], because polyolefin materials are tremendously important
in daily life [6]. Branched polyolefins are generally produced by
transition-metal catalyzed copolymerization. The physical properties of
polyolefin materials can be dramatically affected by their
microstructures, which are controlled by the catalyst structures and
their catalytic behavior. Progress of molecular the catalysts of
transition metal complexes during these three decades enabled control of
stereochemistry of poly(α-olefin)s, control of molecular weight of
polyethylene and poly(α-olefin)s, synthesis of block copolymers by
living polymerization, and copolymerization with various comonomers
including polar functionalized olefins [5]. Thus, the design and
synthesis of novel transition metal catalyst has always been a research
focus of the polyolefin research.
In 1950s, Ziegler & Natta discovered that the
mixture of titanium chloride and alkyl aluminum compounds enabled
polymerization of ethylene and propylene, the synthesis of High Density
Polyethylene (HDPE) and isotactic polypropylene, respectively. The
discovery of [7] catalysts (Figure 1) not only revolutionized polyolefin
production, but also catalyzed the development of the entire
organometallic chemistry field.
In 1980s, Kaminsky [8] discovered that Methyl
Aluminoxane (MAO) as an excellent co-catalyst to highly activate
half-metallocene’s [8] and badged metallocene catalysts for alkene
polymerization to produce polymers of narrow molecular weight
distribution. The second milestone in alkene polymerization catalysis
was the development of metallocene catalysts.

In 1990s, Bwren reported that the constrained
geometry catalysts (CGC, Figure 1) [9] exhibited their unique properties
in the catalysis and revealed the relationship between the structure of
the catalysts and stereoselectivity of the reaction, and showed
high activity for ethylene and 1-alkene copolymerization. In
addition, Fujita also reported that the bis(imine-phenoxy) titanium
and zirconium complexes (FI catalysts, Figure 1) exhibited high
activity for ethylene polymerization [10].
In 1995, Brookhart [11] discovered that nickel and palladium
complexes with bulky diimine ligands showed high catalytic
activity for ethylene and α-olefin polymerizations [11] to produce
high molecular weight polyethylene with different polymer
topologies and branched poly(α-olefin)s [12-13]. Interestingly,
these nickel and palladium catalysts are also used for
copolymerization of ethylene with polar monomers to afford the
copolymers possessing functional groups in a single step because
of their good tolerance toward polar functionality [14,15].
The discovery of late transition metal nickel and palladium
catalysts has initiated a new olefin polymerization field. In
1998, Gibson [16] and Brookhart [17,18] reported that Fe
and Co catalysts with bis (imino) pyridine catalyzed ethylene
polymerization efficiently to afford linear polyethylene. In 2000,
Grubbs [19] reported that neutral nickel catalysts [19] containing
salicylaldimine ligand are used for polymerization of ethylene. In
2002, Pugh [20] reported that palladium catalysts with phoshine
sulfonate ligand can produce unique polymers such as a highly
linear copolymer of ethylene and alkyl acrylates [20] and a nonperfectly
alternating copolymer of ethylene and CO [21].
In this review, recent progress on late transition metal
catalysts for ethylene or α-olefin polymerizations are summarized
[1]. Systematical study elucidated the effects of late transition
metal ion, α-diimine backbone and the ligand on it, the effects
of polymerization conditions (temperature and pressure) on the
catalyst’s performance, branching degree, molecular weight and
its distribution of the polymer and the properties of the polymer
produces.
Chain-walking ethylene polymerization by nickel and palladium catalysts
The research of the ethylene polymerization catalyzed by late
transition metal catalyst should be highlight for development of
polyolefin materials in recent years [22-28], because polyethylene
is probably the polymer you see most in daily life. There are three
main types of polyethylene namely: Low Density Polyethylene
(LDPE), Linear Low-Density Polyethylene (LLDP) and High-
Density Polyethylene (HDPE).
Chain-walking ethylene polymerization mechanism
The discovery of nickel and palladium α-diimine catalysts
(Figure 2) by Brookhart et al. [11] in the 1990s is another further
major advance in the alkene polymerization catalysis [11-13].
Distinctly different from other catalysts, this unique series of late
transition metal catalysts is featured with their characteristic
chain-walking mechanism and allow the unprecedented one-step
synthesis of polyethylenes with controllable chain topology [29-
33].

The nickel and palladium α-diimine catalysts C1 and C2
(Figure 2) showed high activity for polymerization to produce
different branched polymers, depending on the catalyst structures
and the polymerization conditions (temperature and ethylene
pressure), because the the ability of the propagating metal-alkyl
species to migrate along the growing polymer chain via rapid
β-hydride elimination and reinsertion (chain-walking, (Figure 2)
[34-37]. The formation of the major types of branches including
the methyl, ethyl, propyl, butyl, sec-butyl and longer chains, in the
branched polyethylene [36,38]. The mechanism of chain-walking
for ethylene polymerization is shown in (Figure 3).
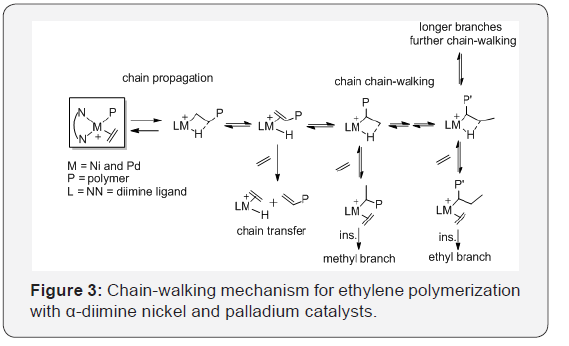
The degree of the polyethylene branching can be controlled via
polymerization conditions [1,39] as follows. For example, Guan et
al. [40,41] demonstrated that ethylene pressure has been used to
control the competition between monomer insertion and chainwalking
processes for ethylene coordination polymerization
catalyzed by a palladium α-diimine catalyst C2. The topology of
the polyethylene changed from linear polyethylene with moderate
branches at high ethylene pressures to a hyperbranched
polyethylene at low pressures.
Modified nickel and palladium catalysts
Previous studies showed that sterically bulky ligands
are usually required to afford nickel and palladium catalysts
capable of generating high activity, stability and high molecularweight
polymers [42-48]. Besides, linear polyethylene via chain
straightening mechanism is the most abundantly produced plastic
owing to its inexpensive monomer, thermoplastic properties,
and semi-crystalline nature [1]. The degree of the polyethylene
branching can be controlled via catalyst structures and
polymerization conditions. For example, Schmid et al. [49] reported
a new “Ortho-Aryl Esffect” using terphenyl substituted 1,4-diaza 1,3-butadiene ligands: the corresponding nickel complexes C3
were highly active catalysts for ethylene homopolymerization to
produce almost perfectly linear polyethylene’s in the presence of
hydrogen [49].
Guan et al. [50-52] designed the macrocyclic ligand by
connecting the aryl substituents of 2,6-diarylphenylimino
moieties using ethylene tether. Their cyclophane-based nickel
and palladium catalysts C4 showed significantly higher thermal
stability than the acyclic analogs [49-52]. The palladium catalysts
also generated polymers with significantly high branching
density compared to the acyclic counterparts, suggesting faster
chain-walking process [50]. The cyclophane-based nickel catalyst
promoted living polymerization of propylene even at 50–75°C [51].
This type of catalyst enhances both the 2,1-insertion of propylene
and the chain-walking reaction. The branching density of the
generated Polypropylene (PP) is slightly above 100/1000C, which
is only half of the value comparing with conventional α-diimine
nickel catalyst. Besides, the palladium catalyst incorporated a
much higher amount of Methyl Acrylate (MA) than the acyclic
analogue (Figure 4).

Inspired by these works, we recently reported a series of
α-diimine nickel and palladium complexes C5 [53,54] containing
2,6-diphenyl groups. These nickel complexes exhibited high
catalytic activity in the polymerization of ethylene (up to 106g PE/
(mol Ni h bar)) and produced highly-branched polyethylenes at
high temperature upon activation with Et2AlCl. The introduction
of an electron-withdrawing group to the ligand framework
improves the catalytic activity significantly. We also reported a
series of α-diimine nickel complexes [35,37,42] containing paraphenyl
or para-naphthyl substituents for ethylene polymerization
under low ethylene pressure. These nickel complexes also
exhibited high catalytic activity and produced highly-branched
polyethylenes.
Brookhart et al. [55] reported a “sandwich” α-diimine nickel
catalyst C6a incorporating two 8-p-tolylnaphthylimino groups
which provide exceptional shielding of the two axial sites
were shown to produce highly branched polyethylene (up to
152 branches/1000C) [55] with ultra-high-molecular-weight.
Recently, they also studied a “sandwich”-type diimine palladium
catalyst C6b for ethylene polymerization, the catalyst conducted
living polymerization at 25°C and produced hyperbranched
polymers [56] (Figure 5).

Coates et al. reported that the precision chain-walking
polymerization of higher α-olefins using the “sandwich” α-diimine
nickel catalyst C6c [57] and some derivative catalysts to afford
highly “chain-straightened” semicrystalline polymers (Tm >
100°C) with a significant fraction of 1, ω-enchainments. We
have reported that a similar naphthyl-α-diimine nickel catalyst
C7 bearing chiral bulky sec-phenethyl groups in the o-naphthyl
position, activated by Et2AlCl [58], showed highly catalytic
activity for the polymerization of ethylene (2.81 × 106 g PE (mol
Ni h bar) −1) and produced branched polyethylene. Interestingly,
C7 could Produce Syndiotactic PMMA at low temperature (rr up
to 88.75%, -30°C).
Conclusion
In this review, the progress on transition metal catalysts
for olefin polymerization were summarized. We first briefly
discussed the chain walking mechanism of nickel and palladium
catalysts in ethylene polymerization. Subsequently, some recent
advances in the α-diimine-based nickel and palladium catalysts
development were summarized. Specifically, the modifications on
N-aryl substituents were described.
Acknowledgements
This work was supported by the National Natural Science
Foundation of China (NSFC, 21801002), and the Natural Science
Foundation of Anhui Province (No. 1808085MB47).
For more articles in Academic Journal of Polymer
Science please click on:
https://juniperpublishers.com/ajop/index.php
https://juniperpublishers.com/ajop/index.php
Comments
Post a Comment