Comparative Study on Polyamide 6 Toughness using Multiple Melt-Kneading Techniques-Juniper Publishers
Authored
by Hiroshi Ito
Abstract
In the present work, we tried to toughen polyamide 6
(PA6) by blending a small amount of low molecular-weight polyethylene
(LMWPE). We obtained the PA6/LMWPE blends with different morphologies
using multiple melt-kneading methods such as the uniaxial melt-kneading,
the eight-axial screw melt-kneading, and the high shearing method. As a
result, it was elucidated that the LMWPE was homogeneously and finely
dispersed by using the eight-axial melt-kneading method. On the other
hand, we found that for high shearing method the LMWPE was finely
dispersed to a degree close to the compatible system. The result of the
three-point bending test showed that the PA6 was brittle fractured, but
it changed to ductile fracture when a small amount of LMWPE was blended.
Also, the fracture displacement was extensively improved. The results
of the comparison by the melt-kneading methods revealed that the
toughness of the PA6 was improved even by adding a small amount of LMWPE
(about 2%) when they were blended using the eight-axial screw
melt-kneading machine. The cross-sectional observation in the bending
test showed that voids were produced from the LMWPE. We elucidated that
the production of voids was induced with low stress by adding fragile
LMWPE, resulting in the improvement of the bending toughness. Moreover,
we found that the LMWPE needs to be finely dispersed with an appropriate
particle size in order to exhibit ductile properties.
Keywords:Polymer blend; Higher order structure; Kneading extruder; High-shear rotational processing; High speed impact test Abbrevations: PA6: Polyamide 6; LMWPE: Low Molecular-Weight Polyethylene; TM: Melting Point; TG: Glass Transition Temperature; MFR: Melt Flow Rate; MW: Molecular Weight; HSP: High-Shear Process Kneader; OSE : Octa Screw Kneading Extruder
Introduction
In recent years, due to the rise in environmental
consciousness, weight reduction aimed at reducing the fuel consumption
of automobiles is proceeding. Under these circumstances, the proportion
of using light polymer in automobiles is increasing. However, extremely
high mechanical properties are required for materials loaded in
automobiles. For that reason, the number of cases has been increasing
where a single type of polymer material cannot meet requirements in
automobiles. One of the methods to solve this problem is to use polymer
blend, a mixture of different kinds of polymer materials. Up to now, the
research and development on polymer blend method have been extensively
conducted as a method that exhibits various properties and functions. As
a result, many polymer alloy materials with various characteristics
have been proposed [1,2]. In particular, for polymer materials showing
brittle fracture behavior, it is tried to change them to be ductile by
blending elastomer and gum. However, with these methods, the rigidity of
the polymer materials is generally reduced while increasing ductility
[3,4].
A crystalline polymer, polyamide 6 (PA6), is an
engineering plastic that has extremely high mechanical property because
of the strong molecular-molecular interaction of hydrogen bonds in amide
group. Although PA6 has strong rigidity, it causes brittle fracture
when imposed with a large displacement. Hence it is very important to
change the property of PA6 to be ductile while maintaining its high
rigidity [5]
In the present study, we tried to improve the
toughness of PA6 by blending a small amount of low molecular-weight
polyethylene (LMWPE). The purpose of the work is to clarify the relation
between the inner structure and the mechanical property of the polymer
blends with different morphologies produced by three different kinds of
melt-kneading methods.
Experimental Part
Samples
PA6 (T802, Toyobo Co., Ltd.) was used as the matrix
resin. The glass transition temperature (Tg) and the melting point (Tm)
of PA6 were 50 °C and 218 °C, respectively. The melt flow
rate (MFR) was 26g/10min at 230 °C. As a modifier of PA6,
LMWPE (Hi wax 1105A, Mitsubishi Chemical Co.) was used. The
LMWPE was denatured by maleic anhydride. The Tg was 104
°C, and the average molecular weight (Mw) of the LMWPE was
1,900. The molded film of LMWPE is an extremely brittle and
low-strength material exhibiting a tensile strength of 1.4MPa
and a breaking strain of 0.006 in a tensile test. The blend ratio
of the samples were PA6/LMWPE=98:2 and 95:5 wt%, and the
kneading temperature was 250 °C.
Melt-kneading methods
The blend ratio, the details of the melt-kneading machines,
and the kneading conditions for the present samples are shown
in Table 1. The melt-kneading machines used were Single screw
extruder (SSE) (CER40, Hoshi Plastic Co., Ltd.), Octa screw
kneading extruder (OSE) (Technobell Co., Ltd.), and High-shear
process kneader (HSP) (Niigata Machine Techno CO., Ltd). The
OSE was used for low-shear kneading. Therefore, it is expected
that we obtain the products with relatively large domain diameter.
The OSE is a machine where all eight screws engage and rotate in
the same direction. Since the resin stays among eight screws in
the OSE, the long-time kneading is possible compared with the
SSE. The HSP is a batch-type kneading machine and has a special
screw with a small hole in the center (return hole). Hence, it is
possible to knead a certain amount of resin at arbitrary rotation
speed and time [6-9].
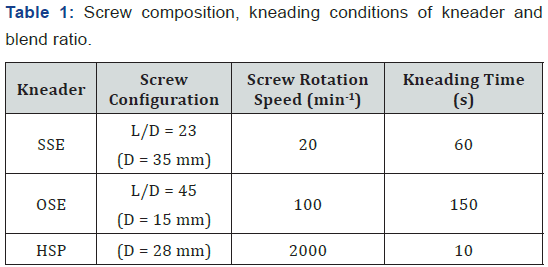
The samples after kneading were pelletized, and then molded
to three-point bending test pieces and flat plate test pieces using
the injection molding machine.
SEM observation
The samples after kneading were frozen and fractured
in liquid nitrogen, and the fracture surface was observed by a
scanning electron microscopy (SEM).
Mechanical properties and observation of plastic deformation region
Three-point bending tests were conducted for specimens
with notches. The Poisson contraction at the tip of the notch is
suppressed due to the constraint of the distortion. Hence the
pure uniaxial stretching test becomes possible. This test method
is known to be effective for the evaluation of toughness (ductile
or brittle) for polymer blend materials [10]. As additional
experiments, the bending test was stopped at the displacement
just before the fracture or at the maximum displacement (10mm),
and the sample was fixed by embedding with epoxy resin.
The plane of the sample perpendicular to the tip of the notch
was then scraped to 20ÎĽm thick thin film using a microtome.
The void-producing region in the plane was observed with a
polarization microscope.
Impact resistance evaluation
For evaluating the impact strength at the time of rapid deformation,
a high-speed impact test (punching) was conducted. The
sample molded to a plane shape was punched by a test rod of
10mm diameter, and the stress was measured. The speed of the
impact test was 10m・s-1.
Results and Discussion
Morphology observation
Figure 1 shows the cross-sectional SEM images for PA6/
LMWPE kneaded by SSE (a) and OSE (b). For the SSE-kneaded
sample, LMWPE aggregates with a maximum particle size of 3ÎĽm
were observed. The size of the aggregates was not homogeneous.
For the OSE-kneaded sample, we confirmed the LMWPE
aggregates with a particle size from 1ÎĽm to 2ÎĽm. The size of the
aggregates was relatively homogeneous compared with that of
the SSE-kneaded sample. For the HSP-kneaded sample, LMWPE
aggregates were not observed. The reason would be that the
LMWPE was extremely finely dispersed in this case.
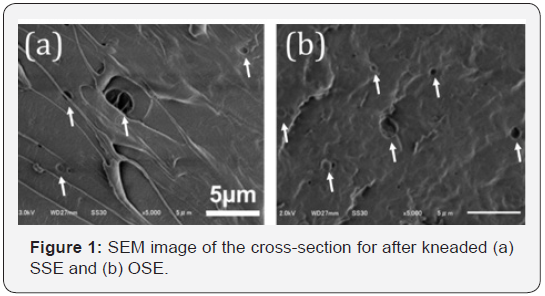
Three point bending test and observation of plastic deformation region
Figure 2 displays the results of the three-point bending test
for pure PA6 and PA6/LMWPE blends produced by respective
kneading methods. Only OSE kneaded-samples were prepared
with LMWPE addition amount of 2 and 5wt%. For the pure
PA6, SSE-kneaded sample, and HPS-kneaded sample, they were
finally brittle fractured. In particular, for the HSP-kneaded
blend, the fracture behavior was same as that of the pure PA6,
thus the effect of the LMWPE blending could not be confirmed.
On the other hand, the OSE-kneaded sample showed plastic
deformation, and it was not fractured even at the maximum
displacement (10mm) resulting in the general yield. The OSE
kneaded sample (LMWPE: 5wt%) showed better breaking
elongation than the system in which LMWPE(added 2wt%),
but the maximum bending strength greatly decreased. This
result seems to be due to excessive addition of brittle LMWPE. Figure 3 presents the polarization microscope image of the
plastic deformation region produced at the tip of the notch in
the bending test. For the pure PA6 (a), voids were not produced
even at 3.2mm deformation, just before the fracture. While
for SSE-kneaded blend (b), the plastic deformation region
originating from the voids formation was confirmed for the
sample just before the fracture (displacement: 6mm). In this
case, the blending of LMWPE induced the void formation, and
the Poisson contraction among the voids became possible, which
led to the ductile behavior of the sample compared with the pure
PA6. However, the stress was locally concentrated because of the
inhomogeneous size of the dispersed LMWPE. Therefore, it is
considered that the sample finally resulted in the brittle fracture.
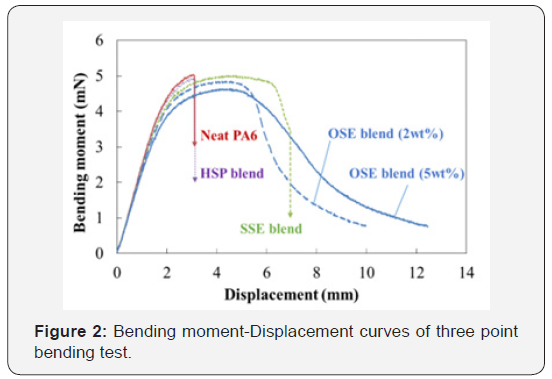
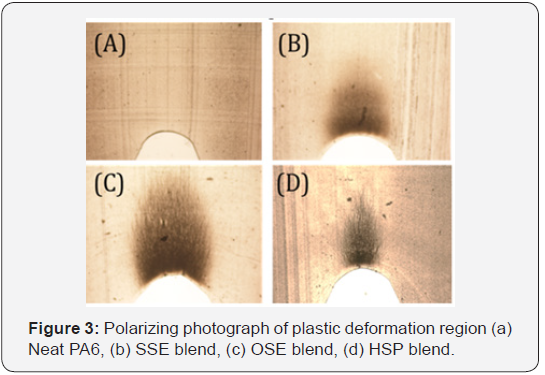
For the OSE-kneaded sample (c), we confirmed the plastic
deformation regions that are wider than those for the SSEkneaded
one at the same displacement (6mm). Compared
with the SSE-kneaded sample, the OSE-kneaded one exhibited
morphology where the size of the LMWPE aggregates was small
and homogeneous. Therefore, the distance among the LMWPE
aggregates was homogeneous, so the sample was homogeneously
deformed due to the dispersion of the stress. As a result, it is
considered that the sample resulted in the general yield without
the brittle fracture even at the maximum displacement.
For the HSP-kneaded sample (d), we observed a fine plastic
deformation region at the displacement (3.2mm), just before
the fracture. By the high shearing kneading, the LMWPE showed
the dispersion condition that is close to the homogeneous
compatibility system. As a result, voids expansion did not proceed
because the size of the produced voids was extremely small.
Thus, the stress constraint was not relaxed because the Poisson
contraction was not sufficient. This would be the reason that
the HSP-kneaded sample finally resulted in the brittle fracture.
Based on the above results, we controlled fragile LMWPE to
exhibit fine (about 1ÎĽm size) and homogeneous higher-order
structure. By doing so, the void formation was induced, and the
stress was relaxed. As a result, we succeeded in improving the
toughness of PA6. On the other hand, the HSP-knead blended
sample with excessively finely dispersed LMWPE did not have a
higher-order structure. We consider that the blending of LMWPE
did not lead to improve the toughness of PA6 in this case because
the stress relaxation function did not work.
High-speed impact test
Figure 4 shows the load-displacement curve in the highspeed
impact test at 10m.s-1. At this speed, both the PA6 and
the HSP-kneaded samples were brittle fractured. The reason
is that in both samples the cracks were developed because the
stress was not relaxed in the deformation at the high speed.
On the other hand, the SSE- and OSE-kneaded samples showed
ductile fracture. For these samples, the LMWPE that is easy to be
fractured existed as domains with 1-3ÎĽm diameter. The stress
relaxation effect expressed even at high speed of 10m・s-1 because
the LMWPE became the origin of voids. Based on these results,
we succeeded in leading the SSE- and OSE-kneaded blends to
the ductile fracture. On the other hand, the effect of the stress
relaxation effect in the HSP-kneaded sample that has extremely
fine dispersed structure of the LMWPE was not sufficient. We
consider this is the reason why the HSP-kneaded sample led to
the brittle fracture.
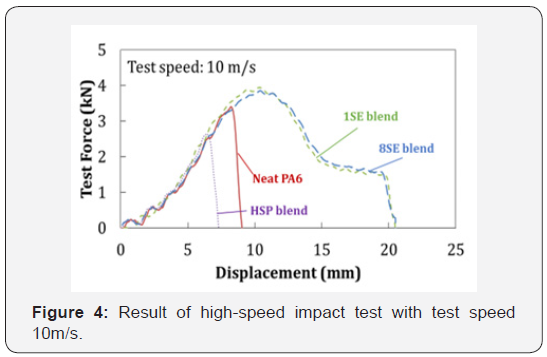
Conclusion
In the present work, we tried to toughen PA6 by
blending a
small amount of LMWPE. We obtained the PA6/LMWPE blends
with different morphologies using multiple melt-kneading
methods such as the uniaxial melt-kneading, the eight-axial screw
melt-kneading, and the high shearing method. As a result, it was
possible to lead to ductile fracture while maintaining rigidity by
adding a small amount of LMWPE to PA6. Kneading by OSE was
effective for finely dispersing LMWPE and equalizing dispersed
particle size, and the properties of the blended material were
also the most stable. This result seems to be because Poisson
contraction was possible even at high speed deformation, and
PA6 could lead to ductile fracture, since LMWPE which breaks
easily is the starting point of void formation. However, when
LMWPE was dispersed in a region close to the nano order and
homogeneous system, the effect of adding LMWPE did not
appear. It has been found that it was important to form a higher
order structure having an island phase size larger than a certain
level for toughening by polymer blending.
Acknowledgement
This research was partially funded by Impulsing Paradigm
Change Through disruptive Technologies (ImPACT) Program of
Council for Science, Technology and Innovation (Cabinet Office,
Government of Japan).
For more
details Journal of Polymer Science please
click on: https://juniperpublishers.com/ajop/index.php
To read more…FullText in Juniper Publishers click on https://juniperpublishers.com/ajop/AJOP.MS.ID.555563.php
Comments
Post a Comment